Introduction
Cholangiocarcinoma (CCA) is an adenocarcinoma characterized by differentiation of the bile duct epithelium. Based on its anatomical location, CCA is classified into intrahepatic CCA (iCCA), perihilar CCA (pCCA), and distal CCA (dCCA) [1,2]. CCAs located more peripherally than the second confluence of the bile ducts are classified as iCCA, those situated between the second confluence and the cystic duct insertion site on the common bile duct are categorized as pCCA, and those found distal to the cystic duct insertion are classified as dCCA [3]. iCCAs account for 10% to 20% of all CCAs, while pCCAs (50% to 60%) and dCCAs (20% to 30%) are more common [3]. However, while the rates of pCCA and dCCA are decreasing, the age-standardized incidence of iCCA has been rising globally over the past few decades, necessitating closer attention [3]. iCCA is the second most common primary liver cancer after hepatocellular carcinoma (HCC) and has a worse prognosis than HCC [4]. Recently, our understanding of iCCA has improved, and it is now recognized as a heterogeneous tumor with diverse etiology, clinical presentation, pathology, and genetic characteristics. The clinical significance of this heterogeneity is being increasingly recognized.
iCCA often presents as a mass with variable shapes, including lobulated or irregular contours, and may be associated with bile duct dilatation, vascular encasement, and regional lymph node metastasis [5]. The enhancement patterns observed on dynamic CT or MRI are diverse; typically, peripheral enhancement is evident in the arterial phase, followed by centripetal enhancement in later phases [6,7]. On MRI, most iCCAs exhibit high signal intensity on T2-weighted images relative to the surrounding liver parenchyma, display diffusion restriction on diffusion-weighted images, and appear hypointense in the hepatobiliary phase when gadoxetic acid is used as a contrast medium [6–8]. The imaging characteristics of iCCA are heterogeneous, reflecting the recently recognized diversity of the disease. Accumulating evidence suggests that these imaging features can be clinically applied to predict prognosis and guide treatment decisions [9,10].
Ethics statement
As this study is based on a review of the literature, neither institutional review board approval nor informed consent was required.
Histopathologic subclassification of intrahepatic cholangiocarcinoma
In the fifth edition of the World Health Organization classification, updated in 2019, a new histological subclassification of iCCA was introduced, delineating small duct (SD-iCCA) and large duct (LD-iCCA) types [11,12]. SD-iCCA and LD-iCCA are distinct not only in their histopathological morphology but also in their etiology, tumor location, gross morphology, histopathological characteristics such as invasiveness and vascularity, molecular features, and prognosis (Table 1).
iCCA, intrahepatic cholangiocarcinoma; PSC, primary sclerosing cholangitis; BilIN, biliary intraepithelial neoplasia; IPNB, intraductal papillary neoplasm of the bile duct; NCAM, neural cell adhesion molecule; N-cadherin, neural cadherin; IDH, isocitrate dehydrogenase; FGFR2, fibroblast growth factor receptor 2; KRAS, Kirsten rat sarcoma viral oncogene homolog; MVD, microvessel density.
SD-iCCA typically arises in the small bile ducts within the peripheral liver, often in the context of chronic hepatitis and cirrhosis. It is characterized by an exclusively mass-forming (MF) gross morphology [13]. Histologically, SD-iCCA consists of cuboidal or low columnar cells arranged in a tubular or cord-like glandular pattern. Compared to LD-iCCA, SD-iCCA is less frequently associated with aggressive pathological features, such as perineural invasion, vascular invasion, and lymph node metastasis [14]. It also tends to have a higher microvascular density (MVD) and exhibits necrosis less frequently. Overall, SD-iCCA has a more favorable prognosis than LD-iCCA [15,16].
LD-iCCA typically originates in the large bile ducts near the hilum and is often associated with underlying chronic bile duct diseases such as hepatolithiasis, liver fluke infestation, or primary sclerosing cholangitis [13]. LD-iCCA frequently arises from a multistep carcinogenesis process, with well-known precursor lesions including biliary intraepithelial neoplasia and intraductal papillary neoplasm of the bile duct [17]. It usually develops from either periductal-infiltrating or intraductal-growing tumors of the large bile duct [14]. These tumors can further evolve into the MF type or present as a hybrid of the MF and either the periductal-infiltrating or the intraductal-growing type [18]. Compared to the SD-iCCA, LD-iCCA more frequently exhibits perineural invasion, vascular invasion, and lymph node metastasis; it also displays lower MVD and more frequent necrosis [14,19]. LD-iCCA has a poorer prognosis than SD-iCCA due to its high invasiveness, which leads to frequent recurrence after curative surgical resection and resistance to chemotherapy [20,21].
Utilizing imaging findings to predict prognosis in intrahepatic cholangiocarcinoma
The prognosis of iCCA in clinical settings is generally stratified using the eighth edition of the American Joint Committee on Cancer (AJCC) staging system. This system incorporates the tumor, node, metastasis (TNM) classification [22] and was developed and validated through pathological assessment of tumor involvement [22–25]. In iCCA staging, the T category is defined by several factors, including the size of the tumor (with a threshold of 5 cm), the number of tumors present, vascular invasion, visceral peritoneal perforation, and invasion of extrahepatic organs. The N category signifies the presence of regional lymph node metastasis, while the M category indicates distant metastasis. Although TNM staging should be definitively determined through pathological examination of resectable tumors, imaging-based staging is commonly employed to predict prognosis and guide treatment decisions for tumors prior to surgical resection or those deemed unresectable.
The eighth edition of the AJCC provides brief guidance on the clinical TNM classification for iCCA, noting that both contrast-enhanced CT and MRI are valuable for detecting tumors larger than 2 cm and assessing vascular involvement. Additionally, it indicates that magnetic resonance cholangiopancreatography can offer additional insights into the extent of disease. However, recent clinical practice guidelines from the European Association for the Study of the Liver and the International Liver Cancer Association (EASL-ILCA) recommend MRI over CT for the staging of iCCA [2]. This guidance is supported by a multicenter retrospective study that directly compared these modalities and revealed the superiority of MRI in staging MF-iCCAs, especially for tumors classified as T1b, T2, and T3/T4. Specifically, MRI displayed better performance in predicting T category components, such as tumor multiplicity, vascular invasion, and visceral peritoneal invasion [26].
The eighth edition AJCC staging system for iCCA categorizes solitary tumors as T1a or T1b, depending on whether the tumor is larger or smaller than 5 cm. This classification is underpinned by multiple studies demonstrating that tumors exceeding 5 cm are linked to comparatively poor survival outcomes and a high likelihood of recurrence [27–29]. Recent research has confirmed the reliability of preoperative imaging for accurately estimating iCCA tumor size, revealing a median difference of less than 0.5 cm between pathological and radiological measurements [30]. Furthermore, a study comparing the effectiveness of CT and MRI for determining iCCA tumor size reported no significant difference between these modalities [26].
Tumor multiplicity is another key determinant for the T category in the AJCC staging system, with its presence categorizing a tumor as T2. Multiplicity is defined as the presence of satellitosis, multifocal tumors, or intrahepatic metastasis [22]. Studies have demonstrated that tumor multiplicity is linked to poorer overall survival and a higher risk of tumor recurrence after resection [31,32]. As previously noted, a recent study showed MRI to be superior to CT in the imaging assessment of tumor multiplicity [26].
In both CT and MRI dynamic imaging studies, the assessment of tumor size and the detection of satellite nodules in iCCA typically involve the use of the portal venous phase [26,33]. Regarding MRI, hepatobiliary phase and diffusion-weighted imaging are also useful, particularly for detecting small lesions [34,35].
Vascular invasion, which includes both macrovascular invasion and microvascular invasion (MiVI) observed on histopathologic examination, is a major prognostic factor for iCCA. Specifically, it assigns a stage of T2 in the eighth edition AJCC system [22]. Research indicates that MiVI in iCCA is significantly linked to poor overall survival. Additionally, macrovascular invasion has been associated with comparatively low overall and disease-free survival [36,37].
The challenge of clinical staging arises from the inability to directly observe MiVI using CT or MRI. To address this issue, recent research has focused on identifying imaging characteristics that can predict the presence of MiVI in iCCA. Studies have found that MiVI is more commonly associated with larger tumors, lobulated or irregular tumor morphology, thin-rim enhancement during the arterial phase, penetration of the hepatic artery within the tumor, bile duct dilation, and a high apparent diffusion coefficient (ADC) [38–40].
Lymph node metastasis is widely recognized as a strong prognostic factor for iCCA [31,41]. The conventional criteria for detecting lymph node metastasis on CT and MRI generally involve a lymph node size threshold of 1 cm in short-axis diameter, along with imaging characteristics such as round shape, irregular margins, and internal necrosis [42]. Recent studies have demonstrated that the inclusion of tumor imaging factors—such as arterial phase hypoenhancement, tumor multiplicity, bile duct involvement, periductal infiltrating growth pattern, and a primary tumor located in the left lobe—combined with serum tumor markers like elevated carcinoembryonic antigen and carbohydrate antigen 19-9 (CA19-9) levels, can improve the accuracy of lymph node metastasis detection in iCCA [43–46].
However, the diagnostic accuracy of CT and MRI for detecting lymph node metastases is generally considered to be inadequate. The most recent EASL-ILCA guidelines advise the routine use of 18-fludeoxyglucose positron emission tomography-CT (18F-FDG PET-CT) for patients with apparently resectable iCCA to achieve precise clinical nodal staging [2]. This recommendation stems from a recent meta-analysis, which revealed that MRI has a sensitivity of 64% and a specificity of 69% for identifying lymph node metastases. In contrast, 18F-FDG PET-CT demonstrated a sensitivity of 52% but a notably higher specificity of 92% [47].
Subclassifying iCCA based on radiological findings can be useful for predicting prognosis, as LD-iCCA is associated with a poorer prognosis than SD-iCCA. Imaging can be employed to assess the differences in gross morphology between these types of iCCA. SD-iCCA typically exhibits a MF appearance with a round or lobulated shape (Figs. 1, 2) [48]. In contrast, LD-iCCA often displays irregular contours alongside a round or lobulated shape, frequent bile duct involvement that is readily apparent on T2-weighted MRI, and vascular encasement (Fig. 3) [9,10,48]. Recent studies indicate that features such as infiltrative contours, adjacent bile duct dilatation, the absence of arterial phase hyperenhancement, and vascular invasion are associated with LD-iCCA and are correlated with poorer disease-free and overall survival [48,49].
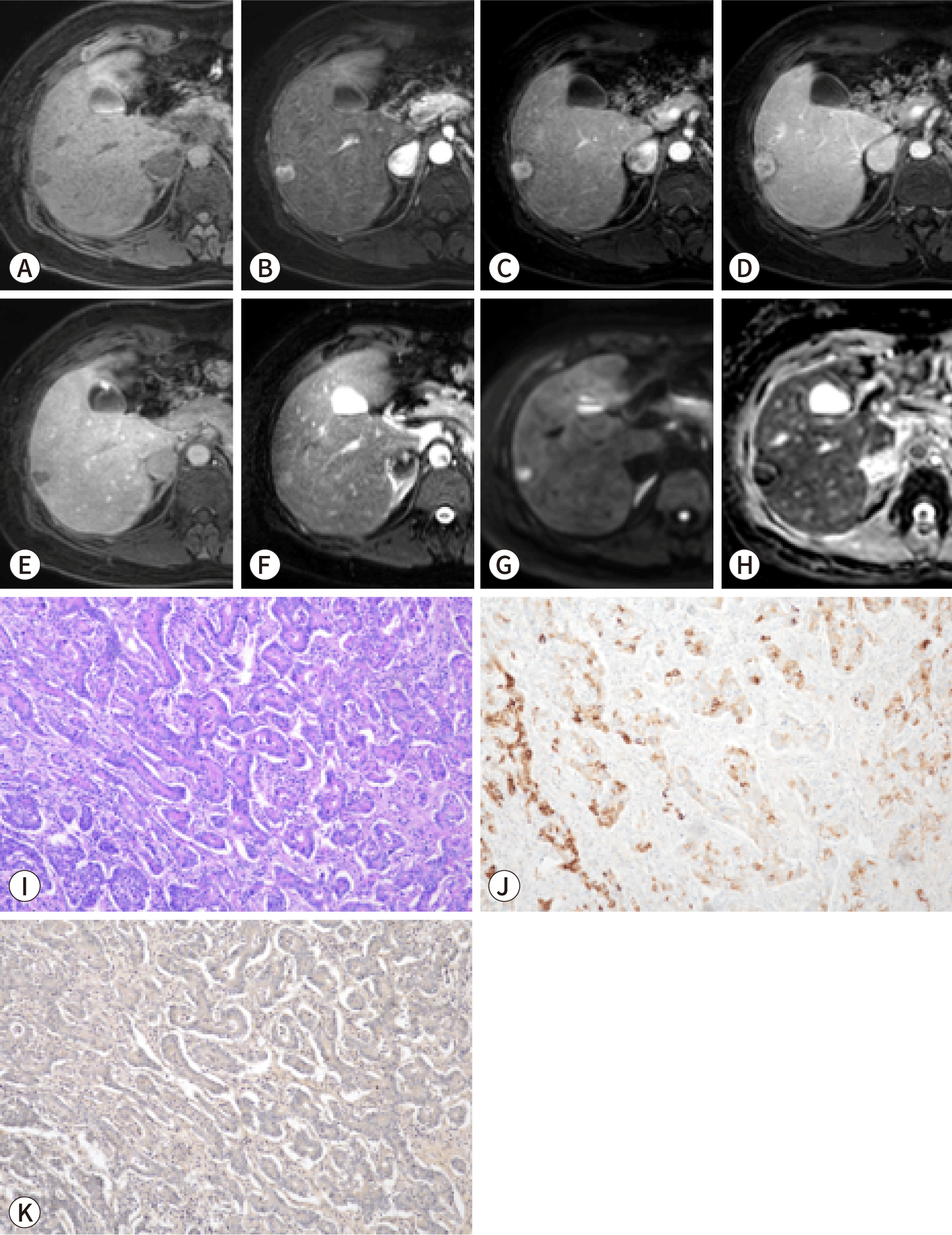
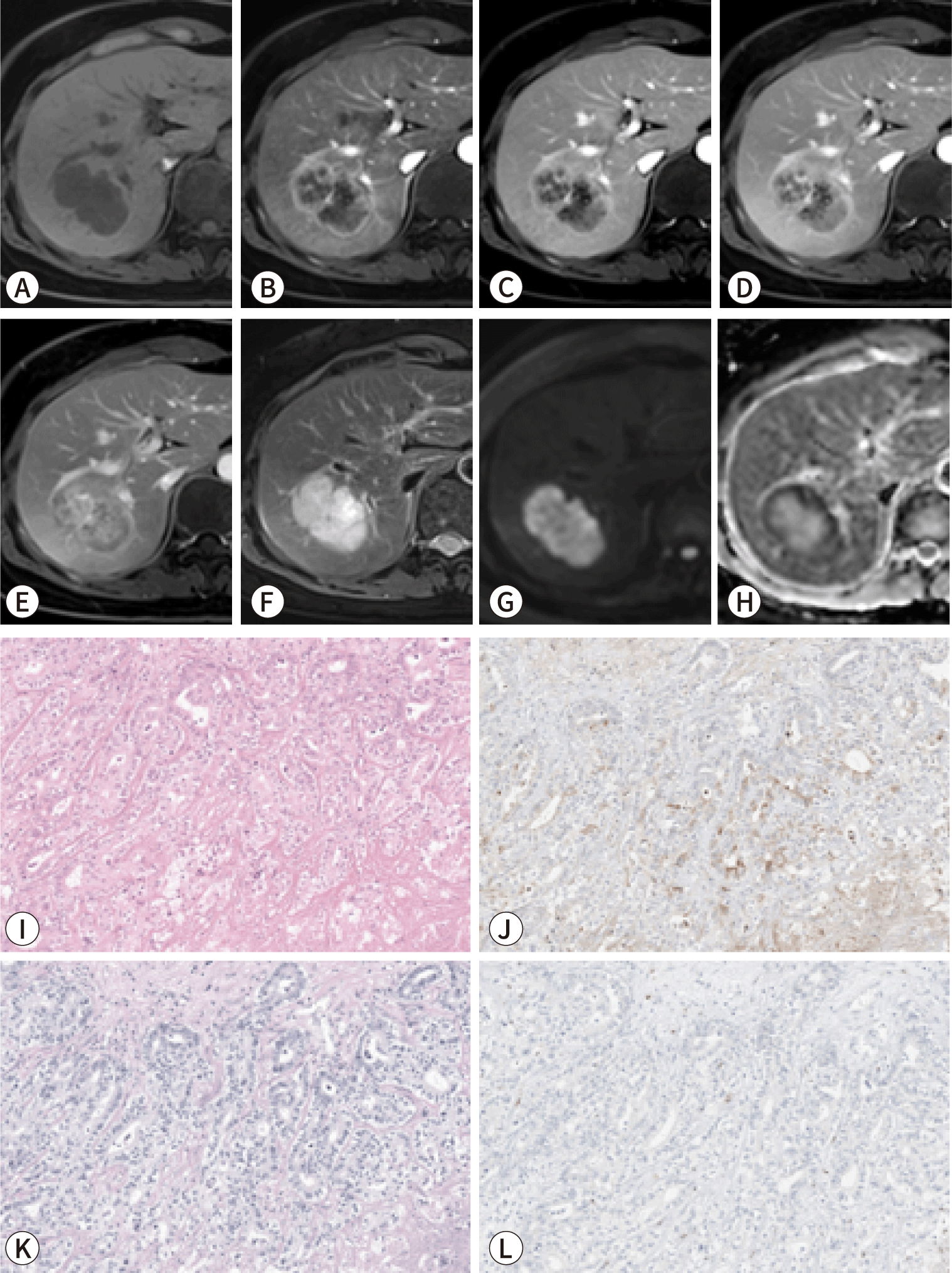
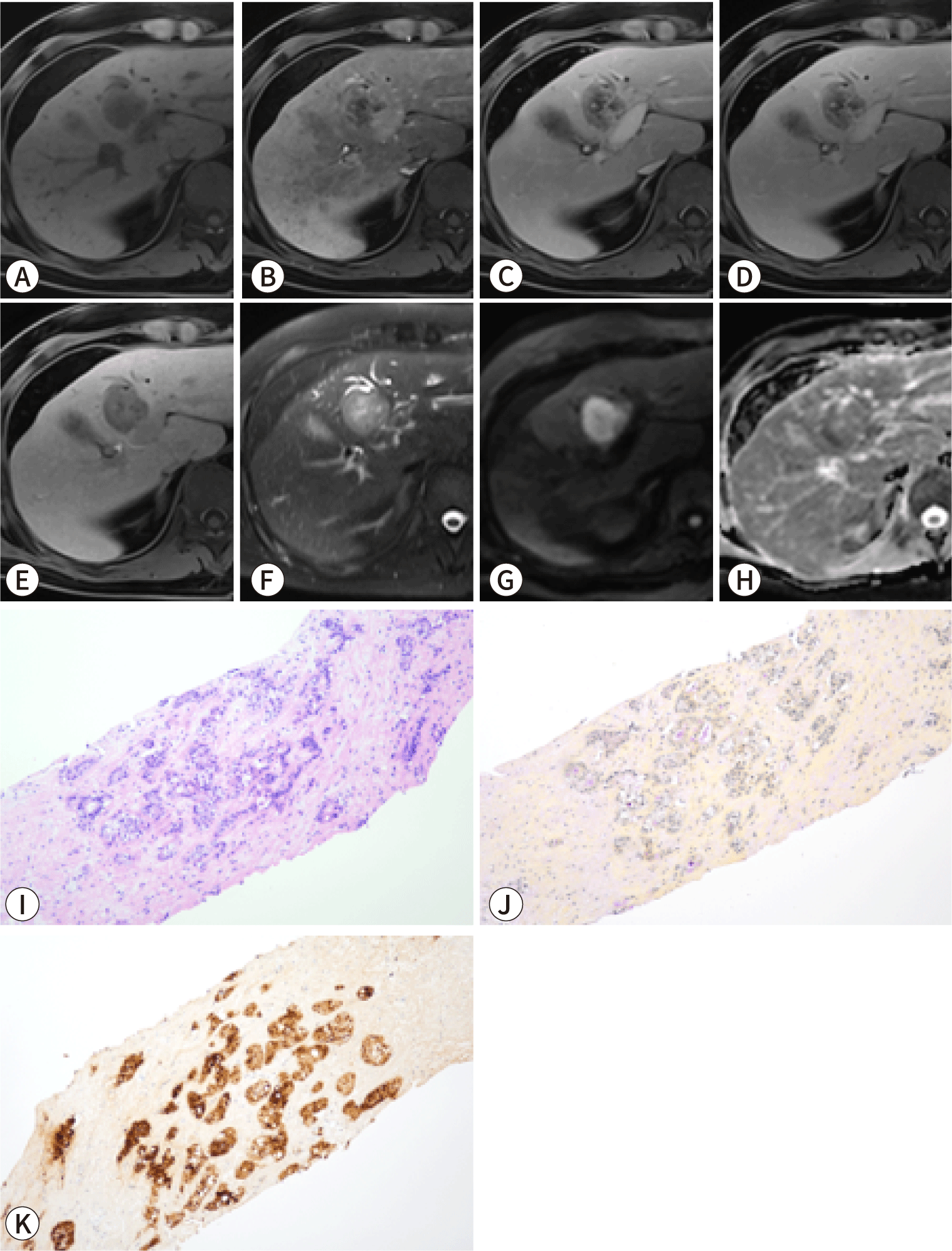
The imaging findings of the two types of iCCA closely align with their pathogenesis and associated liver or bile duct diseases. SD-iCCA is frequently accompanied by chronic hepatitis or cirrhosis, whereas LD-iCCA is commonly associated with chronic bile duct diseases such as primary sclerosing cholangitis, hepatolithiasis, or Clonorchis sinensis infection [50–53].
The arterial enhancement pattern of iCCA is recognized as a key imaging-based prognostic marker. Studies have shown that iCCA with arterial phase hyperenhancement is associated with less invasive histopathological features and better overall survival compared to iCCA with either diffuse hypoenhancement or rim enhancement during the arterial phase (Fig. 1) [54–56]. Radiopathologic correlation studies have additionally established that MVD is linked to arterial enhancement patterns. iCCA with low MVD typically displays low arterial phase enhancement on imaging; furthermore, low MVD is associated with a poor prognosis, along with aggressive pathological features such as tumor multiplicity, MiVI, lymph node metastasis, and low infiltration of CD8+ tumor-infiltrating lymphocytes [57,58].
Moreover, the enhancement pattern of iCCA is suspected to be associated with its subclassification. SD-iCCA often exhibits arterial hyperenhancement, resembling that seen in HCC or combined hepatocellular-CCA [59]. The arterial enhancement patterns in LD-iCCA are more variable, ranging from diffuse hyperenhancement to rim hyperenhancement and diffuse hypoenhancement [48].
Most iCCAs exhibit some degree of fibrous stroma. When scirrhous fibrous stroma comprises more than 70% of the tumor area, the iCCA is classified as scirrhous. Scirrhous iCCA is linked to a higher incidence of lymphatic and perineural invasion and is associated with significantly worse survival outcomes compared to non-scirrhous iCCA [60].
Intratumoral fibrosis can be assessed using dynamic CT or MRI. Radiopathologic correlation studies have demonstrated that regions of delayed enhancement are indicative of contrast retention within the fibrous stroma of the tumor [61–63]. Furthermore, the degree of delayed enhancement has been recognized as a prognostic marker for poor outcomes in patients with MF-iCCA. Specifically, iCCAs that exhibit delayed enhancement in more than two-thirds of the tumor on dynamic CT are associated with scirrhous iCCA, a higher incidence of perineural invasion, and relatively low overall survival [64].
Similarly, on gadoxetic acid-enhanced MRI, central intratumoral enhancement observed during the hepatobiliary phase reflects the presence of fibrous stroma within an iCCA lesion (Fig. 4) [65,66]. During this phase, iCCAs containing fibrous stroma exhibit an ill-defined hyperintense region set against a peripheral hypointense area. This pattern is often described as the “EOB-cloud,” a term derived from the chemical name of gadoxetic acid, gadolinium ethoxybenzyl diethylenetriamine pentaacetic acid (Gd-EOB-DTPA) [66,67]. This cloud-like appearance results from the extracellular accumulation of contrast in the central fibrous stroma, while the periphery of the tumor typically contains a higher proportion of tumor tissue and less fibrosis. Notably, MF-iCCAs in which more than 50% of the area appears hyperintense or isointense during the hepatobiliary phase tend to exhibit comparatively poor disease-free and overall survival [68].
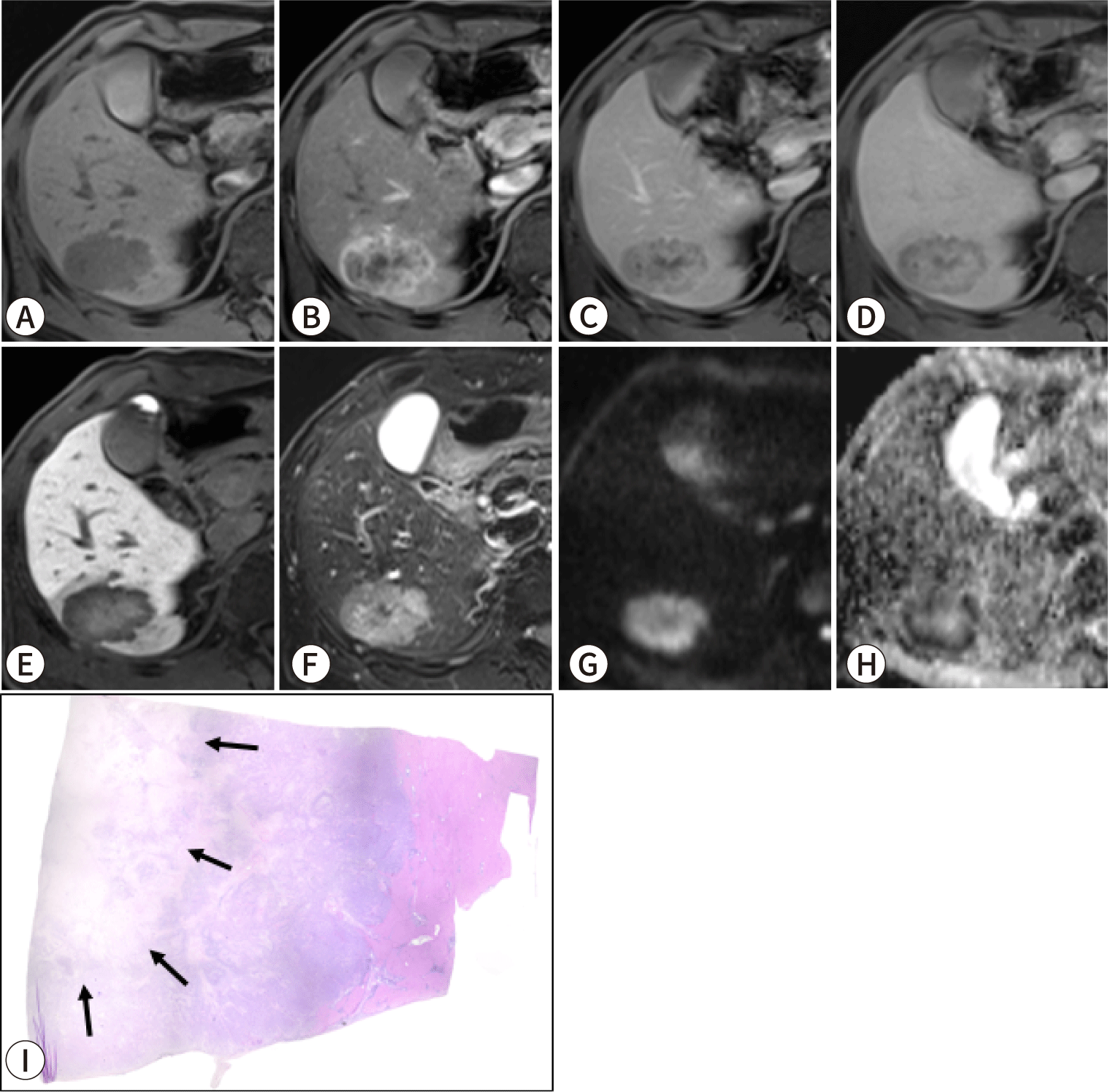
Recent research has progressed from correlating imaging findings with histopathologic characteristics associated with prognosis to creating models that directly predict prognosis from imaging findings. These imaging-based prognostic models can offer insights into patient outcomes before treatment is initiated, potentially informing critical decisions about therapeutic strategies.
A multicenter study proposed a preoperative prognostic model for resectable iCCA that includes serum CA19-9 levels and three MRI findings: tumor multiplicity, lymph node metastasis, and bile duct invasion [69]. In predicting overall survival, this imaging-based model demonstrated comparable discriminatory performance to traditional pathologic staging systems, such as the eighth edition AJCC TNM system, the MEGNA score, and Nathan staging [69–71]. Another recent study introduced a more sophisticated prognostic model for resectable iCCA, combining two serum markers (CA19-9 >300 IU/mL and albumin ≤40 g/L) and six imaging findings [72]. This model outperformed pathologic staging systems, including the eighth edition AJCC and the MEGNA score, in predicting disease-specific and disease-free survival [72].
Imaging-based models have also been reported for predicting the prognosis of unresectable iCCA. The Fudan score, originally developed from a cohort of patients with resectable iCCA, has been shown to be effective for prognostic prediction in unresectable iCCA as well [73]. This score includes five variables: tumor diameter, the number of intrahepatic tumors, the type of tumor boundary, serum alkaline phosphatase levels, and CA19-9 levels. A recent study proposed a modified scoring system for unresectable iCCA by incorporating an additional unfavorable prognostic factor—high ADC—which improved the model’s performance in predicting survival [74].
Utilizing imaging findings for treatment decision-making in intrahepatic cholangiocarcinoma
Imaging is vital in the treatment decision-making process for iCCA, as it aids not only in assessing resectability but also in determining the need for lymphadenectomy during hepatic resection, deciding whether to administer neoadjuvant therapy, and selecting targeted therapies.
Lymphadenectomy plays a crucial role in accurate staging and may reduce the risk of recurrence; however, its impact on survival remains unclear. Although the practice of lymph node dissection in patients with clinically positive lymph nodes (cN+) has gained broader acceptance, the routine dissection of lymph nodes in patients who lack clear evidence of lymph node metastases (clinically negative lymph nodes, cN–) remains a topic of ongoing debate [75,76].
The AJCC staging system, EASL-ILCA guidelines, and National Comprehensive Cancer Network (NCCN) clinical practice guidelines all endorse the routine dissection of lymph nodes. The AJCC and EASL-ILCA guidelines specifically recommend the removal of a minimum of six lymph nodes to ensure thorough nodal staging. In comparison, the NCCN guidelines simply advises regional lymphadenectomy of the porta hepatis [2,22,77]. Conversely, Japanese guidelines do not provide a specific recommendation concerning routine lymph node dissection [78].
In clinical practice, lymph node dissection is performed in about half of patients, largely based on the surgeon’s discretion [79,80]. When deciding whether to perform lymph node dissection, imaging findings play a key role. One study revealed that a risk score combining serum carcinoembryonic antigen level (≥7 ng/mL), lymph nodes deemed suspicious on MRI, and MRI evidence of bile duct invasion was significantly correlated with the presence of pathological lymph node metastasis (Fig. 5) [46]. Moreover, among patients who had a high risk score but did not undergo lymph node dissection, the researchers observed a higher likelihood of nodal recurrence within 3 months after surgery [46]. Imaging results can therefore be instrumental in stratifying the risk of lymph node metastasis, thus guiding the decision of whether to perform lymph node dissection in patients at high risk.
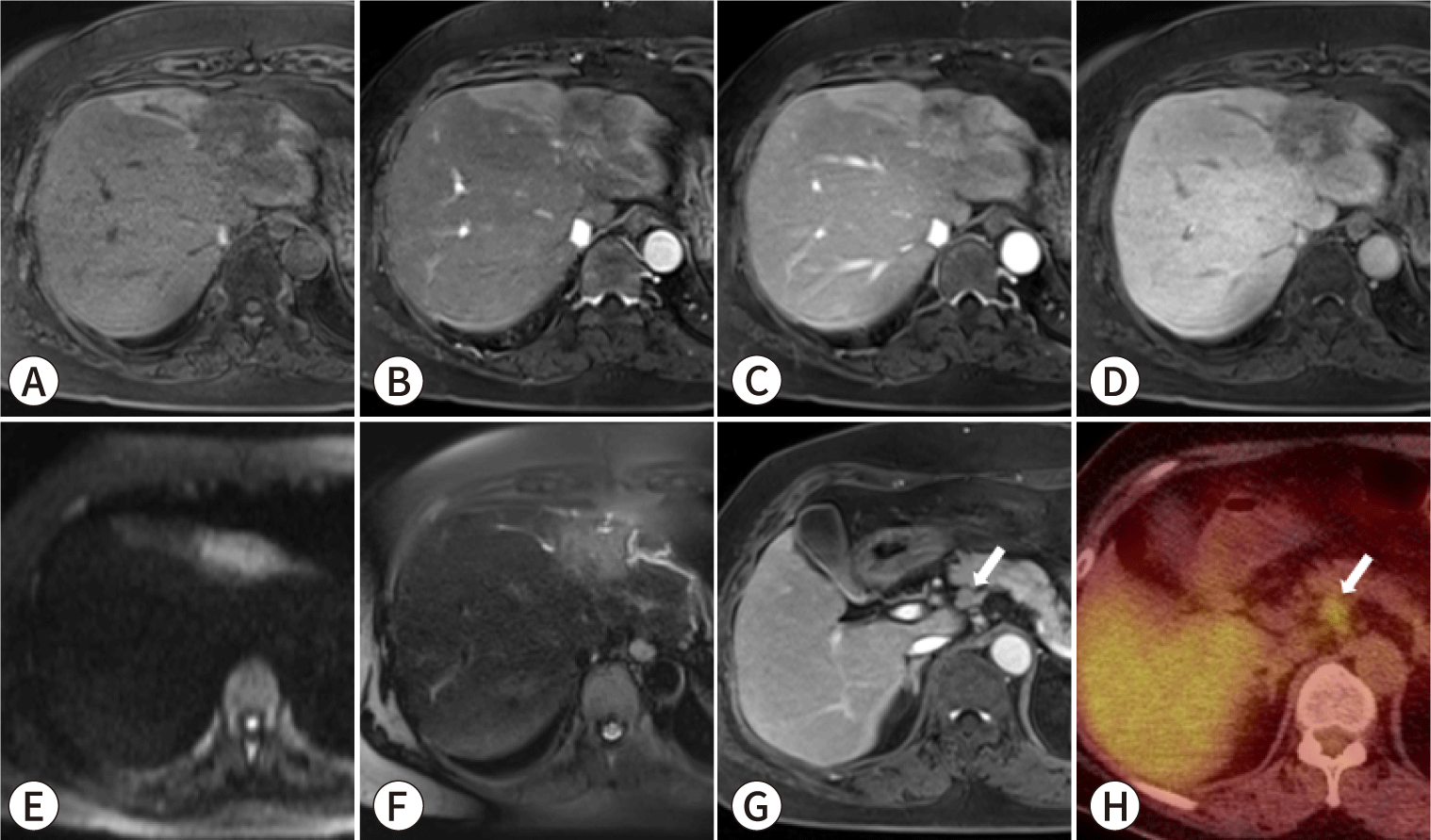
Currently, the literature includes no prospective evidence specifically supporting neoadjuvant therapy for iCCA, and no randomized studies have directly compared neoadjuvant chemotherapy followed by surgery with surgery alone. However, two retrospective studies, both analyzing data from the US National Cancer Database, yielded notable findings.
One study demonstrated that patients with a higher clinical T stage or clinical lymph node metastasis who received neoadjuvant therapy had better survival outcomes than those who underwent upfront surgery [81]. Similarly, another study found that although no survival benefit was observed in a propensity score-matched analysis across all stages of iCCA, neoadjuvant therapy did confer a survival advantage in patients with more advanced disease (stages II-III) [82].
As a result, guidelines vary in their recommendations concerning neoadjuvant therapy for iCCA. The EASL-ILCA guideline states that neoadjuvant systemic chemotherapy can be considered for patients with surgically challenging yet resectable disease, especially when an R1 resection is anticipated [2]. In contrast, neither the NCCN nor the Japanese guidelines provide specific recommendations for neoadjuvant therapy in the context of iCCA [77,78]. Well-designed prospective trials are necessary to further evaluate the role of neoadjuvant treatment in this context.
Collectively, neoadjuvant therapy has been demonstrated to confer a survival benefit in cases with a high T stage, suspected nodal metastasis, or a high likelihood of positive resection margins. Considering that imaging-based prognostic models are highly effective at predicting postoperative outcomes, the use of imaging to identify high-risk patients who might benefit from neoadjuvant therapy could represent a viable strategy [72].
In recent years, targeted therapy has become increasingly important in the treatment of iCCA [83]. The standard first-line treatment consists of a combination of gemcitabine, cisplatin, and durvalumab. However, targeted therapies are emerging as a viable second-line option for patients with specific genetic mutations [2,77]. These therapies require molecular profiling, often performed through next-generation sequencing, which can be expensive. Molecular profiling is typically recommended for patients with advanced-stage disease who need systemic therapy and for early-stage patients at high risk of recurrence. Imaging findings can be instrumental in identifying and stratifying these high-risk patients.
Currently, approved targeted therapies are available for genetic factors including isocitrate dehydrogenase 1 (IDH1) mutation, fibroblast growth factor receptor 2 (FGFR2) fusion, BRAF V600E mutation, microsatellite instability-high and mismatch repair-deficient cancers, and epidermal growth factor receptor 2 (HER2) overexpression [84–88]. Among these, IDH1 mutation and FGFR2 fusion are particularly impactful due to their comparatively high incidence [77]. These mutations are predominantly observed in SD-iCCA [18]. Studies have shown that iCCA with IDH1/2 mutations often presents with pronounced arterial phase enhancement on imaging, a feature commonly associated with SD-iCCA [89]. Given the higher prevalence of targetable mutations such as IDH1 and FGFR2 within the small duct type, molecular profiling may be particularly promising when imaging findings suggest the presence of SD-iCCA (Fig. 6). However, limited research is available to support this recommendation.
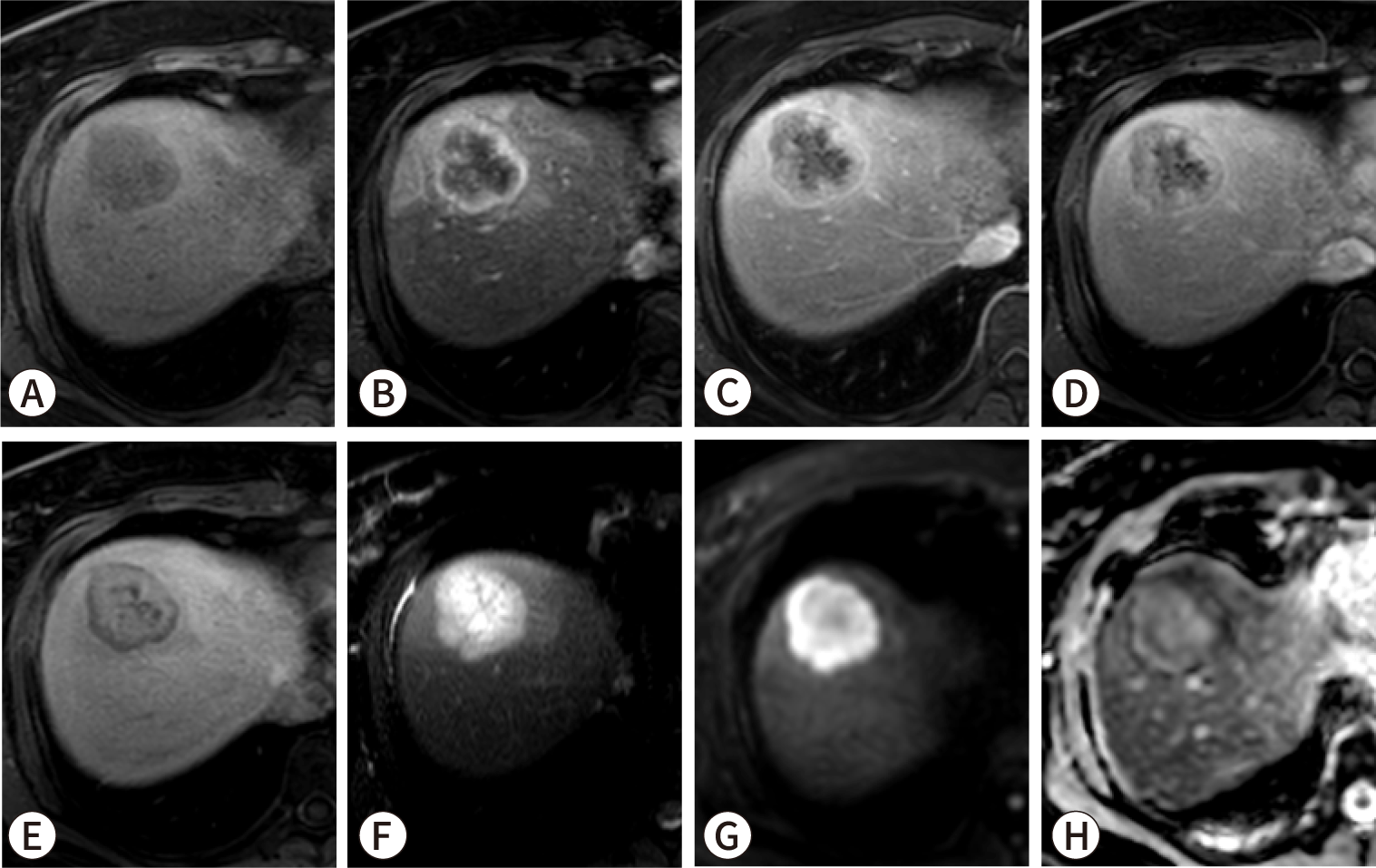
Conclusion
iCCA represents a serious and escalating global health concern, with a rising incidence and persistently poor prognosis. However, advancements in pathology and radiology have provided new insights into the disease. The histological subclassification into SD-iCCA and LD-iCCA offers a valuable framework for understanding the heterogeneity of iCCA and improving prognosis prediction. Radiological studies that focus on various imaging findings, such as tumor size, multiplicity, enhancement patterns, the presence of intratumoral fibrous stroma, and suspicious lymph nodes, have demonstrated high utility in the pre-treatment assessment of patients.
Importantly, imaging-based prognostic models for resectable iCCA have demonstrated predictive accuracy comparable to that of traditional pathological staging systems. Beyond predicting prognosis, imaging also offers critical insights that may inform decisions about lymphadenectomy and neoadjuvant therapy. Furthermore, radiological findings indicative of SD-iCCA could assist in identifying patients likely to harbor clinically relevant mutations, such as IDH1 mutatios and FGFR2 fusions.
These imaging-based approaches are essential for improving prognosis and tailoring treatment strategies for patients with iCCA, thereby advancing personalized medicine in this area. Nevertheless, to increase the utilization of imaging findings in predicting prognosis and informing treatment decisions, higher-level evidence from international multicenter prospective studies is necessary.