Introduction
Primary liver cancer ranks as the third leading cause of cancer-related deaths globally, with nearly 1 million new cases reported annually [1]. Hepatocellular carcinoma (HCC) accounts for approximately 90% of all primary liver cancers. It typically arises in the setting of chronic liver diseases, which may be due to HBV, HCV, alcohol-related liver disease, or metabolic dysfunction-associated steatotic liver disease (MASLD) [2]. MASLD is estimated to affect around 20%–25% of the global population [3]. Metabolic dysfunction-associated steatohepatitis (MASH) is characterized by more than 5% steatosis, hepatocellular injury (such as "ballooning"), and inflammation, which may occur with or without fibrosis [4]. About 20% of individuals with MASLD develop MASH, which is strongly linked to rising rates of obesity, diabetes, and metabolic syndrome. As MASH progresses, it can lead to severe liver-related complications, including cirrhosis or liver failure, and significantly increases the risk of developing HCC [5].
In patients with MASH-related cirrhosis, the annual incidence of HCC is approximately 2% [6]. Moreover, MASH is the primary cause of HCC in patients who do not have cirrhosis [7]. MASH-related HCC accounts for 20% of HCC cases in the Western world and is projected to become the leading cause of HCC globally by 2030 [8]. The development of MASH-related HCC is characterized by unique mutational, immunological, and microenvironmental features. Although most cases of MASH-related HCC occur in patients with cirrhosis, 30%–40% develop in those with advanced fibrosis but without cirrhosis. This suggests a distinct metabolic environment and the likely involvement of extrahepatic cancer drivers associated with metabolic syndrome [9,10]. Unlike infections with HBV or HCV, MASH more frequently leads to HCC in the absence of cirrhosis, underscoring the need for strengthened surveillance and early detection [11].
Currently, MASH-HCC is managed similarly to other causes of HCC, employing strategies such as transplantation, resection, or locoregional therapies for early- or intermediate-stage disease [12]. MASH is the leading cause of HCC-related liver transplants in the USA; however, approximately 50% of patients undergo systemic therapy as their disease progresses, which includes both combination therapies and single-agent treatments with tyrosine kinase inhibitors or monoclonal antibodies [13]. Nevertheless, it remains uncertain whether immune-based therapies are as effective for non-viral HCC as they are for viral-related HCC [14].
In this review, we examine the clinical management of MASH-HCC, focusing on surveillance strategies and recent advancements in treatment. We also discuss the customized application and outcomes of surgical, locoregional, and systemic therapies, examining future prospects and strategies to address current challenges.
Ethics statement
It is a literature database-based review; therefore, neither approval by the institutional review board nor obtainment of informed consent was required.
Epidemiology
Approximately 10% (ranging from 1% to 38%) of all HCC cases are associated with MASLD, with higher rates (>20%) reported in studies from the USA, UK, India, Germany, and the Middle East. In contrast, lower estimates (1%–2%) are reported from China and Japan [15]. The incidence of MASH-related HCC is expected to rise substantially as the obesity epidemic continues to expand [16]. Mathematical models predict a significant increase in the incidence of MASH-HCC from 2016 to 2030, with projected rises of 47% in Japan, 82% in China, 88% in the UK, 117% in France, and 130% in the USA [17]. Compared to patients with HCC due to viral hepatitis (HBV or HCV) or alcohol-related liver disease, those with MASH-HCC typically have a lower male-to-female ratio (1.2:1), are generally 5–10 years older (mean age 73), and are more likely to have metabolic and cardiovascular comorbidities, such as type 2 diabetes mellitus (DM) and chronic vascular disease. Although the incidence of MASH-HCC is lower than that associated with active viral hepatitis, the increasing prevalence of MASLD, combined with improved treatments for viral hepatitis, is expected to increase both the proportion and rate of HCC attributed to MASLD [18,19].
Risk factors
A study involving approximately 300,000 patients with MASLD reported an HCC incidence of 0.21 per 1,000 person-years, which is seven times higher than that observed in control individuals without liver disease—specifically, those free from viral hepatitis and with normal alanine aminotransferase levels [20]. The primary risk factor for MASH-HCC is cirrhosis, with incidence rates in cohorts of MASH cirrhosis estimated at about 2% per year, although these rates vary from 0.3% to 4.7% per year [6]. This variability can be attributed to differences in age, metabolic profiles, and the severity of liver decompensation. While HCC can also develop in MASH patients without cirrhosis, the overall incidence in this subgroup is low, ranging between 0.01% and 0.13% per year. It is even lower in the general MASLD population, underscoring the importance of assessing cirrhosis status as the primary risk stratifier for MASLD [21].
In cohort studies from both Europe and the US, type 2 diabetes has been identified as the strongest independent metabolic risk factor for the development of HCC. A retrospective study demonstrated that in patients with MASH-cirrhosis, the presence of DM was associated with a fourfold increase in the risk of developing HCC (hazard ratio [HR], 4.2; 95% CI, 1.2–14.2; P=0.02) [19]. Another large study in Europe, which included 136,703 patients with MASLD, found that among the 6,425 (4.7%) patients with advanced fibrosis, DM was the most significant risk factor for HCC [22]. Similarly, a study involving a US cohort of 271,906 MASLD patients, of whom 253 had HCC, reported a strong association between DM and HCC (adjusted HR, 2.77; 95% CI, 2.03–3.77) [23].
In a large cohort study involving 296,707 patients, those diagnosed with MASLD and obesity did not show a statistically significant increase in HCC risk (P=0.06). However, the risk increased significantly, by 2.6 times, when obesity was accompanied by diabetes, hypertension, and hyperlipidemia [20]. Another recent study, which examined data from 98,090 MASLD patients with severe obesity, found that those who underwent bariatric surgery experienced a reduced risk of HCC. The adjusted HR was 0.48 (95% CI, 0.24–0.89) [24]. Although numerous studies have explored the link between obesity and elevated HCC risk, most have not sufficiently evaluated the presence of MASLD or MASH.
The impact of mild to moderate alcohol consumption on the development of HCC in patients with MASLD is still unclear, as research has produced inconsistent findings. A cohort study in Korea examined the relationship between mild to moderate alcohol intake and the progression of non-invasive fibrosis scores in 58,927 adults with MASLD who initially had low fibrosis scores over a median period of 4.9 years [25]. Of these participants, 5,303 (9%) progressed from low to intermediate or high fibrosis scores. Moderate drinkers were more likely to experience increased fibrosis compared to nondrinkers, with an HR of 1.29 (95% CI, 1.23). Another study indicated that even mild drinking habits increased the risk of carcinogenesis in patients with MASH-associated cirrhosis, presenting an HR of 3.8 (95% CI, 1.6–8.9; P=0.002); however, this study focused solely on patients with decompensated liver disease [26]. Additionally, a recent multivariate analysis of patients with biopsy-proven MASLD across various stages of fibrosis revealed that consuming less than 20 g of alcohol per day heightened the risk of HCC, especially in those with advanced F3–4 fibrosis, with a relative risk of 4.83 (P=0.04) [27].
Smoking is generally associated with an increased risk of HCC; however, its specific impact on MASLD has not been thoroughly investigated [28].
Coffee is rich in antioxidants, including phenolic compounds such as chlorogenic, caffeic, ferulic, and coumaric acids, along with melanoidins and diterpenes such as cafestol and kahweol. These compounds have shown inhibitory effects on the development of HCC [29,30]. Additionally, the beneficial effects of coffee in preventing HCC may be partially attributed to its role in lowering the risk of type 2 DM, which is a known risk factor for HCC [31].
Metformin inhibits the mammalian target of the rapamycin pathway, which plays a role in cell proliferation by activating AMP-activated protein kinase (AMPK) [32]. It also inhibits angiogenesis, disrupts the cell cycle, and induces apoptosis independently of p53 [33]. Additionally, metformin promotes moderate weight loss, mitigates the effects of hyperinsulinemia on the cell cycle and inflammation, and improves liver biochemistry and histology in patients with MASLD [34,35]. Research has explored the impact of antidiabetic medications on HCC risk, recognizing diabetes as a significant risk factor. A recent study demonstrated that effective glycemic control was associated with a 31% reduced risk of HCC in patients with MASLD and DM [36]. The study also found that metformin use led to a 20% decrease in HCC risk, whereas insulin use, particularly when combined with other oral antidiabetic medications, increased the risk by 1.6 to 1.7 times. However, a database study of 18,080 MASLD patients without cirrhosis, monitored over an average of 6.3 years, showed no link between metformin use and HCC risk [37]. In a recent nationwide cohort, patients with MASLD and DM who used sodium-glucose cotransporter-2 inhibitors had significantly lower risks of liver and non-liver complications compared to users of other antidiabetic medications, with HRs ranging from 0.76 to 0.97. The risk was further reduced when metformin was also used, with HRs between 0.58 and 0.79 [38].
Statins exhibit a range of anticancer effects that go beyond their ability to lower cholesterol. They inhibit key oncogenic drivers including MYC, AKT, Rho-dependent kinase, and extracellular signal-regulated kinase 1 and 2 [35,39,40]. Additionally, statins activate protective liver pathways such as AMPK and p38-MAPK, and promote apoptosis through a p53-dependent mechanism [41,42]. These drugs have also been linked to anticarcinogenic effects. A database study from Taiwan involving 18,080 MASLD patients demonstrated an inverse relationship between statin use and HCC, with an OR of 0.29 (95% CI, 0.12–0.68) [37]. In a retrospective case-control study of 102 MASLD patients, including 34 HCC cases, statins were found to be protective against HCC (OR, 0.20; 95% CI, 0.07–0.60) [43]. Another recent retrospective study showed that statin use significantly and dose-dependently reduced the risk of HCC in patients with NASH cirrhosis [44]. However, a study involving 458 MASLD patients with advanced fibrosis did not find such an association [45]. The uncontrolled and retrospective nature of these studies limits the ability to definitively interpret their findings on the chemopreventive benefits of statins, making it inappropriate to recommend them solely for the prevention of HCC.
Pathogenesis
Approximately 80% of MASLD patients do not develop NASH, prompting research efforts to focus on identifying the factors that differentiate those with inflammation, cell injury, and fibrosis (MASH) from those exhibiting simple steatosis. A critical factor in understanding the progression to MASH is lipotoxicity, which involves hepatocellular injury resulting from disrupted fat metabolism [46]. Lipotoxicity is triggered by various factors, including increased fatty acid delivery to the liver, insulin resistance, and inflammatory signals from dysfunctional adipose tissue [47]. This condition leads to cellular stress, oxidative damage, inflammasome activation, and ultimately, cell death in hepatocytes [48]. These damaging responses are linked to pre-malignant changes, such as oxidative DNA damage and mutations in metabolism-related genes such as FOXO1, CIDEB, and GPAM. Although these genes may help protect hepatocytes from lipotoxicity, they also elevate the risk of malignancy [49,50].
To repair hepatocellular injuries in MASH, developmental pathways such as YAP–TAZ, Notch, and Hedgehog signaling are reactivated in hepatocytes. This reactivation leads to cell proliferation, inflammation, and potentially cancer [51,52]. In advanced MASH, there is a marked decline in hepatocyte proliferation and regenerative capacity. These dysregulated cells exacerbate inflammation and fibrosis [53]. Consequently, this hepatocellular damage fosters a pro-inflammatory environment, perpetuating chronic inflammation and impacting various immune cell types.
The stage of hepatic fibrosis in MASH is a critical determinant of clinical outcomes, as it can progress to cirrhosis and liver failure, and create conditions conducive to cancer development [54]. This process involves the activation or transdifferentiation of resident hepatic stellate cells (HSCs) into fibrogenic, proliferating myofibroblasts, which leads to the accumulation of extracellular matrix or scar tissue. Advanced single-cell sequencing has revealed significant heterogeneity among HSCs in MASH, although the functional implications of this diversity are not yet clear [55]. The exact mechanisms by which MASH-HCC develops without cirrhosis remain poorly understood, but they are likely related to fibrosis. The accumulation of extracellular matrix increases liver stiffness, which can facilitate the emergence and growth of tumor cells [56]. This scar matrix also acts as a reservoir for growth factors that may support the survival of pre-neoplastic hepatocytes, thereby promoting tumor initiation or progression. Additionally, HSCs possess immunoregulatory properties that contribute to the liver's immune tolerance, potentially affecting its response to checkpoint blockade therapies [57].
Angiogenesis is implicated in both MASH and potentially MASH-HCC. Increased CD34 expression in new blood vessels has been observed in previous studies involving both humans and rodents, indicating enhanced vascularization [58]. Vascular endothelial growth factor (VEGF), a crucial angiogenic signal, shows elevated levels in experimental MASH models. Inhibiting VEGF leads to reduced vascularization, inflammation, and steatosis [59].
The impact of treatments targeting MASH on the risk of MASH-HCC has yet to be determined; however, a decrease in HCC risk has been noted in MASH patients following bariatric surgery, indicating that future medical interventions for MASH could potentially lower the incidence of HCC [60]. Nonetheless, it remains uncertain whether advanced liver fibrosis continues to carry an inherent risk of cancer even if the fibrosis subsequently regresses.
The immune system plays a major role in both MASLD and HCC, and distinct immunogenomic classifications have been identified [61]. MASH is characterized by inflammatory responses in the liver, which are pivotal in its progression to fibrosis, cirrhosis, or HCC [62]. Both innate and adaptive immune mechanisms significantly contribute to hepatic inflammation in MASH. Resident Kupffer cells and the recruitment of leukocytes, including neutrophils, monocytes, NK cells, and NKT cells, promote inflammation through the release of cytokines, chemokines, and reactive oxygen species. Elevated levels of CD4+ T helper cells, particularly the TH1 and TH17 subsets, have been observed in the livers of mice with MASH [63]. Although T cells exhibit anti-tumorigenic properties, the depletion of CD8+ T cells accelerates tumor growth in MASH-driven HCC models. Similarly, the depletion of CD4+ T cells promotes tumor growth, impacting the efficacy of immune-based therapies [64].
The disruption of the immune system in MASH and MASH-HCC has been linked to the response to immunotherapies. Both adaptive and innate immune cells, including CD4+ T cells, metabolically activated CD8+ T cells, platelets, and dendritic cells, play a role in shaping the liver microenvironment as MASH progresses to HCC [65,66]. Neutrophils, in particular, are involved in the transition from fatty liver to steatohepatitis. They contribute to an immunosuppressive environment through the production of extracellular traps and PDL1 signaling, which leads to CD8+ T cell exhaustion and affects the response to immunotherapy [67,68]. In MASLD, impaired antigen-specific T-cell function has been observed, partially due to macrophage activity [69]. In advanced HCC, the infiltration of CCR2+ and CX3CR1+ macrophages is linked to non-responsiveness to immune-checkpoint inhibition. Conversely, pro-inflammatory PDL1-expressing CXCL10+ macrophages can drive treatment response. Recent studies indicate that T cells lose functionality in MASLD, which contributes to poor responses to immune checkpoint inhibitor (ICI) therapy [70]. Approaches such as neutrophil reprogramming with CXCR2 antagonists have shown promise in enhancing the effectiveness of ICI therapy in MASH-HCC models by increasing dendritic cell activity and CD8+ T cell numbers [68].
In two notable studies involving both mice and humans, the presence of CD8+PD1+ T cells in the liver increased as MASH progressed. These cells are in an auto-aggressive state, characterized by liver-resident CD8+PD1+CD103+ T cells that, despite being exhausted, display an activated phenotype and express high levels of cytokines such as TNF, CCL2, IL-10, and granzyme B [71,72]. In MASH-HCC mouse models treated with immunotherapy, these CD8+PD1+ cells exhibited minimal changes in their transcriptomes and proteomes, yet they increased in size over time. This growth contributed to heightened liver inflammation, hepatocyte death, and oncogenic signaling [72]. Instead of eliminating HCC, these cells became dysfunctional in tumor surveillance and even promoted tumor growth. This dysfunction resulted in a lack of response to ICIs in therapeutic settings and accelerated HCC development in preventive scenarios. Similar characteristics of CD8+ T cells have been observed in human MASH-HCC, indicating that peritumoral and intratumoral CD8+PD1+ T cells could potentially serve as predictors of treatment success or resistance to ICIs. Understanding the immune microenvironment is essential for identifying the most effective therapies in future research.
The gut microbiome plays a crucial role in influencing altered liver responses in MASH by affecting hepatic bile acid metabolism and facilitating the translocation of gut-derived signals through an increasingly permeable gut lining [73]. Throughout all stages of NASH, the gut–liver axis remains active, with interactions between liver damage, regeneration, and heightened gut permeability exacerbating inflammatory, pro-fibrogenic, and pro-carcinogenic pathways [48]. This permeability defect allows for both direct (e.g., bacterial presence) and indirect (e.g., bacterial metabolites) interactions between the gut microbiome and the liver, which in turn impact liver metabolism and contribute to the progression of MASH and HCC.
The gut microbiome has been identified as a key factor in triggering MASLD, driving liver steatosis by enhancing energy harvest, monosaccharide absorption, and abnormal acetate production [74]. A dysbiotic, leaky gut permits the translocation of pathogen-associated and danger-associated molecular patterns into the liver, activating immune cells and Toll-like receptors, which in turn trigger pro-inflammatory and fibrotic pathways [75]. In mice, disruption of the gut vascular barrier by the microbiota is seen as a precursor to NASH [76]. Additionally, inflammatory cells from the gut may migrate to the liver, contributing to bacterial translocation. Several bacterial species, such as Proteobacteria, Enterobacteriaceae, and Escherichia, are associated with MASLD in humans, and levels of Bacteroides are elevated in MASH patients [76,77]. Treatment with non-absorbable antibiotics, such as rifaximin, has shown potential in improving liver function, underscoring the significant role of the gut microbiome in MASH pathogenesis [78].
Several single-nucleotide polymorphisms (SNPs) associated with abnormal lipid metabolism in hepatocytes have been linked to an increased risk of MASH and progression to HCC. One of the most well-known SNPs is rs738409 in the PNPLA3 gene, which encodes the patatin-like phospholipase domain-containing protein 3. This variant interferes with the breakdown of lipid droplets in hepatocytes, leading to decreased triglyceride lipolysis and promoting hepatic steatosis. As a result, it is associated with more than a 2-fold increased risk of MASH and a 2.2-fold higher risk of progressing to MASH HCC compared to those without the variant [79]. Another significant SNP, rs58542926 in the TM6SF2 gene, plays a role in regulating liver fat metabolism and increases hepatic triglyceride content. This variant is linked to a 1.6-fold increased risk of MASH and a 1.9-fold higher risk of MASH HCC [80].
Additionally, an SNP near the MBOAT7 gene is associated with increased hepatic triglyceride levels and occurs twice as frequently in patients with MASH-HCC compared to those with MASLD alone [81]. A loss-of-function variant in the GCKR gene, which encodes the glucokinase regulator, leads to increased de novo lipogenesis and insulin resistance. This variant is linked to a 1.5-fold increased risk of MASH and a 1.8-fold higher risk of MASH-HCC [82]. A polygenic risk score that incorporates these four SNPs has been suggested for HCC risk stratification in patients of European ancestry with NASH cirrhosis. This score has proven to be a more accurate predictor of HCC development than individual SNPs (P<10–13) [83].
MASH-HCC is often associated with an increased presence of ACVR2A and TP53 mutations, as well as the proliferative class S1-WNT/TGFβ [84]. A distinct mutational signature, termed MutSigNASH-HCC, has been identified in 25% of MASH-HCC patients, compared to only 2% in those with other causes. This signature is characterized by a higher frequency of C>T and C>A transitions [85]. Furthermore, patients with MASH-HCC exhibit higher levels of hepatic oxidative DNA damage than those with other etiologies, a phenomenon that correlates with a diminished DNA damage response in experimental models [49]. Additionally, epigenetic events that suppress the transcription of genes involved in bile and fatty acid metabolism, while activating proliferative pathways, have been implicated in MASH-HCC. Experimental models have shown that epigenetic reprogramming can reverse hepatocarcinogenesis [86].
A diagram of the pathogenesis of HCC associated with metabolic dysfunction-related steatohepatitis is shown in Fig. 1.
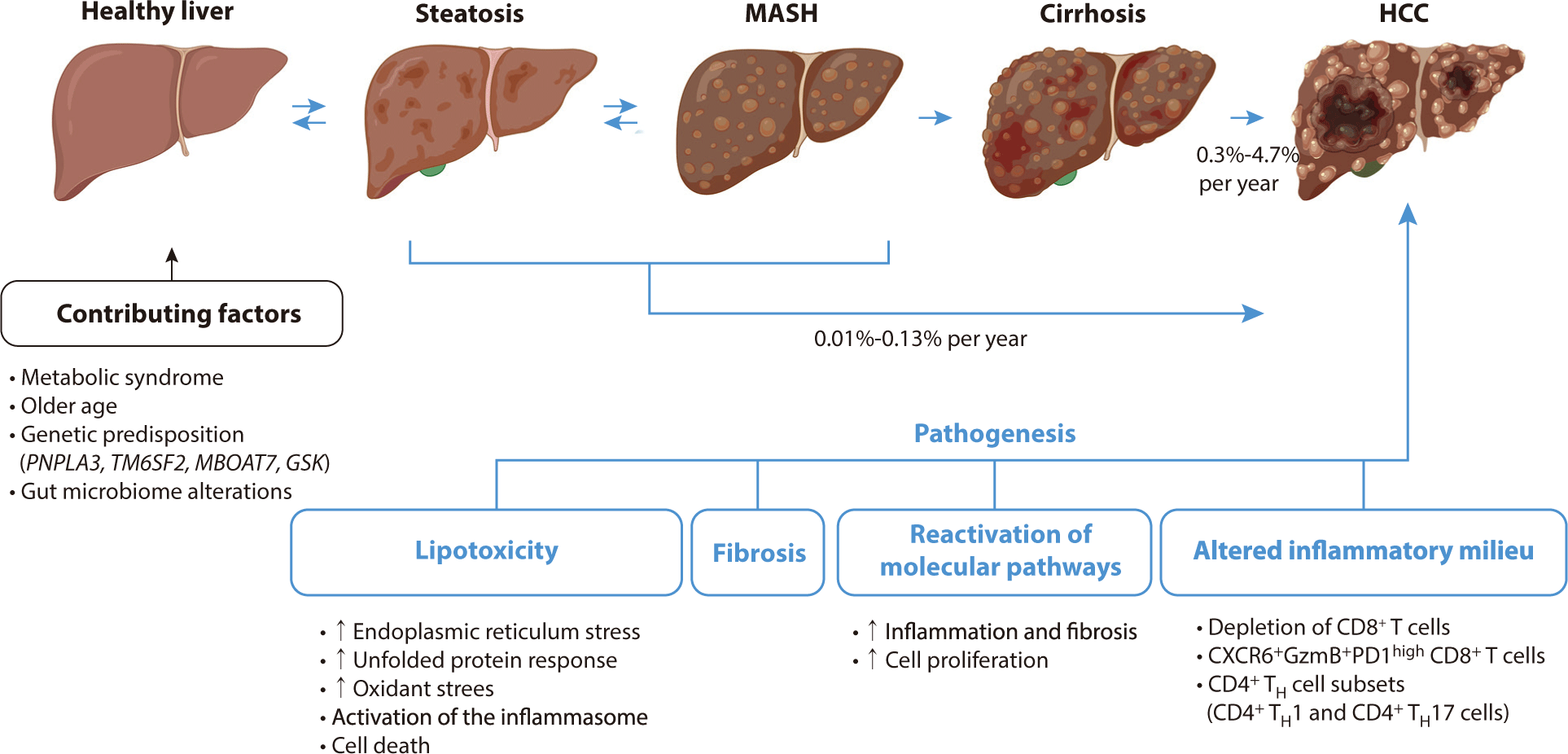
Clinical management
Several observational, retrospective, population-based studies have suggested that metformin, statins, coffee, and aspirin might contribute to the prevention of HCC, regardless of the underlying liver disease etiology [87,88]. Due to its generally favorable benefit-to-risk ratio, current guidelines endorse the consumption of coffee for individuals with chronic liver disease [89,90]. However, other agents have not demonstrated sufficient efficacy to be recommended for HCC prevention, and most studies related to this have not been conducted in well-defined populations with MASLD.
For the prevention of MASH-HCC, the American Association for the Study of Liver Diseases (AASLD), the European Association for the Study of the Liver (EASL), and the Korean Association for the Study of Liver Diseases (KASL) recommend combining a hypocaloric or Mediterranean diet with moderate-intensity exercise to achieve and maintain weight loss, as outlined in their practice guidelines [89–91]. Additionally, a large multinational cohort study has demonstrated that physical activity is associated with a reduced risk of HCC [92]. Although there is no direct evidence currently available that weight loss decreases the risk of MASH-HCC, observational studies indicate that weight loss may reverse steatosis and potentially fibrosis in patients with MASH, thereby suggesting a possible benefit of weight loss in reducing the risk of HCC [93,94].
The clinical practice guidelines from the AASLD, EASL, and KASL recommend semiannual surveillance for HCC using abdominal ultrasound, with or without α-fetoprotein testing, for all patients with cirrhosis, regardless of the underlying cause. However, only two studies have specifically assessed the potential benefits of such surveillance in patients with MASLD-related cirrhosis.
However, a previous study found no significant association between surveillance and the applicability of curative treatment (45.5% versus 51.5%; P=0.72) [95].
Data specifically focusing on patients with MASLD are important, as this group exhibits unique characteristics that pose challenges to traditional HCC surveillance methods. Notably, about one-third of MASLD-HCC cases arise in individuals without cirrhosis, suggesting that these patients are often excluded from the at-risk populations typically targeted for surveillance [21]. Furthermore, at the time of their HCC diagnosis, patients with MASLD are generally less likely to have been previously diagnosed with liver disease or cirrhosis, which likely contributes to their lower rates of surveillance utilization [96]. A meta-analysis revealed that a significantly smaller proportion of patients with MASLD-HCC (32.8%, 95% CI, 12.0–63.7) underwent surveillance compared to patients with HCC from other causes (55.7%, 95% CI, 24.0–83.3; P<0.0001) [97].
Second, patients with MASH are more likely to experience inadequate ultrasound visualization and surveillance failure, leading to a higher rate of late-stage HCC diagnoses even when surveillance is performed [98,99]. This suggests that the sensitivity of ultrasound-based surveillance in patients with MASH may be lower than the 63% observed in those with HCC from other causes [100]. This finding underscores the need for alternative imaging methods, such as CT or MRI, and blood-based biomarker strategies for this group [101].
Patients with MASH-HCC often present with comorbidities, such as cardiovascular disease, which can restrict their access to curative treatments, especially surgery [102,103]. However, a systematic review has shown that despite having more comorbidities and larger tumors at diagnosis, the allocation of treatments for MASLD patients is similar to that for other patients [97]. Moreover, when severe comorbidities are excluded, the outcomes following curative and locoregional treatments for MASH-HCC are comparable to, or even better than, those observed in non-MASH patients. Lastly, immunotherapies may be less effective in non-viral HCC cases, such as MASH-HCC, due to impairments in the immune system [72].
Surgery: Patients with MASLD face a higher risk of intra-operative complications and poorer post-surgical outcomes, largely due to the increased prevalence of metabolic syndrome comorbidities. Obesity and type 2 diabetes have been linked to lower survival rates in cancer patients, including those receiving surgical treatments [104,105]. Research indicates that patients with MASH-HCC are more likely to suffer from hypertension, hyperlipidemia, and ischemic heart disease compared to those with other causes of HCC, all factors that heighten the risk of post-surgical morbidity and complications [102]. Furthermore, the degree of liver steatosis may correlate with poorer surgical outcomes [106].
However, a systematic review and meta-analysis of 14 studies, which included 7,226 HCC patients—approximately 20% of whom had MASH-HCC—demonstrated that patients with MASH-HCC experienced improved disease-free survival (HR, 0.81) and overall survival (HR, 0.78) compared to those with other causes [107]. Another meta-analysis corroborated these results, suggesting that the better outcomes in MASH-HCC patients might be due to the absence of cirrhosis in many cases and the exclusion of those with severe comorbidities from surgical interventions [108].
Liver transplantation: An analysis of the United Network for Organ Sharing (UNOS) registry from 2002 to 2012 revealed that patients with MASH-HCC had significantly better post-transplant survival outcomes (HR, 0.69; 95% CI, 0.63–0.77) and a lower risk of graft failure (HR, 0.76; 95% CI, 0.69–0.83) compared to those with other causes of HCC. This was despite a higher prevalence of diabetes and cardiovascular disease in the MASH-HCC group [109]. In contrast, data from the European Liver Transplant Registry showed no statistically significant differences in post-transplant survival or graft survival between patients with HCC, regardless of MASLD status. However, there were differences in the causes of mortality [110]. While some single-center studies suggest that patients with MASLD may have a higher risk of post-transplant complications, the overall evidence indicates similar post-transplant survival rates between patients with MASLD and those with other etiologies of HCC [111].
Locoregional therapies: Current evidence on the efficacy of locoregional therapies for MASH-HCC is limited. However, a study using the SEER-Medicare database showed similar overall survival rates following radiofrequency ablation in patients with MASH-HCC compared to those with other HCC etiologies [112]. Additionally, a propensity score-matched study that included patients undergoing transarterial chemoembolization revealed no significant differences in time-to-progression (13.0 vs. 8.5 months; P=0.25) or overall survival (23.2 vs. 28.0 months; P=0.48) between patients with and without MASLD [113]. Another study comparing MASLD-HCC and HBV-related HCC patients treated with transarterial radioembolization also found no significant differences in treatment-related adverse events or overall survival [114]. These results indicate that transarterial chemoembolization and transarterial radioembolization are likely safe and effective treatments for patients with MASH-HCC, yielding comparable outcomes across different etiologies.
Systemic therapies: Phase III studies of systemic therapies in advanced HCC have predominantly involved patients with compensated liver disease. However, the etiology of liver disease has not been a consideration in treatment decisions or trial designs. Typically, studies report efficacy based on stratification factors such as etiology, often categorized as HBV, HCV, or “non-viral.” The “non-viral” category includes alcohol-related disease, MASH, and other causes (Table 1).
Trial | Treatment arms | Subgroup based on etiology (n, %) | Overall survival HR (95% CI) | Progression-free survival HR (95% CI) |
---|---|---|---|---|
Immunotherapy: first line | ||||
IMbrave150 [118] | Atezolizumab plus bevacizumab vs. sorafenib |
Overall (501) HBV (240, 48%) HCV (108, 22%) Non-viral (153, 31%) |
0.58 (0.42–0.79) 0.51 (0.32–0.81) 0.43 (0.22–0.87) 0.91 (0.52–1.60) |
0.59 (0.47–0.76) 0.47 (0.33–0.67) 0.69 (0.39–1.20) 0.71 (0.47–1.08) |
COSMIC-312 [119] | Atezolizumab plus cabozantinib vs. sorafenib |
Overall (649) HBV (190, 29%) HCV (202, 31%) Non-viral (257, 40%) |
0.90 (0.69–1.18) 0.53 (0.33–0.87) 1.10 (0.72–1.68) 1.18 (0.78–1.79) |
0.63 (0.44–0.91) 0.46 (0.29–0.73) 0.64 (0.38–1.09) 0.92 (0.60–1.41) |
HIMALAYA [120] | Tremelimumab plus durvalumab vs. sorafenib |
Overall (782) HBV (241, 31%) HCV (214, 27%) Non-viral (327, 42%) |
0.78 (0.65–0.93) 0.64 (0.48–0.86) 1.06 (0.76–1.49) 0.74 (0.57–0.95) |
0.90 (0.77–1.05) - - - |
Durvalumab vs. sorafenib |
Overall (778) HBV (238, 31%) HCV (211, 27%) Non-viral (329, 42%) |
0.86 (0.73–1.03) 0.78 (0.58–1.04) 1.05 (0.75–1.48) 0.82 (0.64–1.05) |
1.02 (0.88–1.19) - - - |
|
CheckMate 459 [121] | Nivolumab vs. sorafenib |
Overall (743) HBV (233, 31%) HCV (173, 23%) Non-viral (336, 45%) |
0.85 (0.72–1.02) 0.77 (0.56–1.05) 0.71 (0.49–1.01) 0.95 (0.74–1.22) |
0.93 (0.79–1.10) - - - |
RATIONALE-301 [122] | Tislelizumab vs. sorafenib |
Overall (674) HBV (427, 63%) HCV (85, 13%) Non-viral (162, 24%) |
0.85 (0.71–1.02) 0.91 (0.73–1.14) 0.64 (0.38–1.08) 0.78 (0.55–1.12) |
1.11 (0.92–1.33) - - - |
LEAP-002 [123] | Pembrolizumab plus lenvatinib vs. lenvatinib |
Overall (794) HBV (385, 47%) HCV (181, 22%) Alcohol (251, 31%) |
0.84 (0.71–1.00) 0.75 (0.58–0.97) 0.86 (0.60–1.24) 0.84 (0.67–1.05) |
0.83 (0.71–0.98) - - - |
CARES-310 [124] | Camrelizumab plus rivoceranib vs. sorafenib |
Overall (543) HBV (405, 75%) HCV (51, 9%) Non-viral (87, 16%) |
0.62 (0.49–0.80) 0.66 (0.50–0.87) 0.45 (0.18–1.16) 0.71 (0.37–1.36) |
0.52 (0.41–0.65) 0.57 (0.45–0.72) 0.46 (0.21–1.05) 0.55 (0.33–0.93) |
ORIENT-32 [125] | Sintilimab plus IBI305 vs. sorafenib |
Overall (571) HBV (538, 94%) Non-HBV (33, 6%) |
0.57 (0.43–0.75) 0.58 (0.43–0.76) 0.80 (0.22–2.87) |
0.56 (0.46–0.70) 0.56 (0.40–0.76) 0.38 (0.14–1.06) |
Immunotherapy: second line | ||||
KEYNOTE-240 [126] | Pembrolizumab vs. placebo |
Overall (413) HBV (101, 24%) HCV (64, 15%) Non-viral (248, 60%) |
0.78 (0.61–1.00) 0.57 (0.35–0.94) 0.96 (0.48–1.92) 0.88 (0.64–1.20) |
0.72 (0.57–0.90) 0.70 (0.44–1.13) 0.46 (0.24–0.90) 0.75 (0.56–1.01) |
KEYNOTE-394 [127] | Pembrolizumab vs. placebo |
Overall (453) HBV (360, 79%) Non-HBV (93, 21%) |
0.79 (0.63–0.99) 0.78 (0.61–0.99) 0.87 (0.53–1.44) |
0.74 (0.60–0.92) 0.77 (0.61–0.98) 0.58 (0.36–0.94) |
Tyrosine kinase inhibitors: first line | ||||
SHARP [128,129] | Sorafenib vs. placebo |
Overall (439) HBV (111, 18%) HCV (169, 28%) Alcohol (159, 26%) |
0.69 (0.55–0.87) 0.76 (0.38–1.50) 0.50 (0.32–0.77) 0.76 (0.50–1.16) |
- - - - |
Asia–Pacific [130,131] | Sorafenib vs. placebo |
Overall (226) HBV (165, 73%) Non-HBV (61, 27%) |
0.68 (0.50–0.93) 0.74 (0.51–1.06) 0.57 (0.29–1.13) |
- - - |
REFLECT [132] | Lenvatinib vs. sorafenib |
Overall (753) HBV (479, 50%) HCV (217, 23%) Alcohol (57, 6%) |
0.92 (0.79–1.06) 0.83 (0.68–1.02) 0.91 (0.66–1.26) 1.03 (0.47–2.28) |
0.66 (0.57–0.77) 0.62 (0.50–0.75) 0.78 (0.56–1.09) 0.27 (0.11–0.66) |
Tyrosine kinase inhibitors: second line | ||||
CELESTIAL [133] | Cabozantinib vs. placebo |
Overall (707) HBV (267, 38%) HCV (168, 24%) Non-viral (272, 38%) |
0.76 (0.63–0.92) 0.69 (0.51–0.94) 1.11 (0.72–1.71) 0.72 (0.54–0.96) |
0.44 (0.36–0.52) 0.31 (0.23–0.42) 0.61 (0.42–0.88) 0.48 (0.36–0.63) |
RESORCE [134] | Regorafenib vs. placebo |
Overall (573) HBV (216, 38%) HCV (119, 21%) Alcohol (145, 25%) |
0.63 (0.50–0.79) 0.58 (0.41–0.82) 0.79 (0.49–1.26) 0.92 (0.61–1.38) |
0.46 (0.37–0.56) 0.39 (0.29–0.54) 0.59 (0.39–0.90) 0.53 (0.37–0.77) |
REACH-2 [135] | Ramucirumab vs. placebo |
Overall (292) HBV (107, 37%) HCV (76, 26%) Other (109, 37%) |
0.71 (0.53–0.95) 0.84 (0.52–1.35) 0.76 (0.44–1.33) 0.63 (0.38–1.06) |
0.45 (0.34–0·60) 0.43 (0.28–0.68) 0.33 (0.19–0.60) 0.57 (0.35–0.95) |
Currently, several agents are approved for the first- and second-line treatment of advanced HCC. These can be broadly categorized into two groups: multi-kinase VEGFR-targeting small molecules and VEGFR2 monoclonal antibody approaches, as well as immunotherapy-based approaches. Regarding overall survival, the efficacy of the first group does not significantly vary based on the etiology of HCC, as evidenced by similar HRs for overall survival in the study versus control arms. This trend is also generally observed in secondary endpoints, such as progression-free survival and objective response rates.
Unlike previous treatments, ICIs have not only demonstrated a survival benefit but have also achieved significant response rates with durable responses lasting over 20 months. There is growing interest in evaluating clinical characteristics as markers of benefit, especially those associated with distinct pathogenic pathways and immune profiles linked to different HCC etiologies. Two studies have raised questions about the effectiveness of immunotherapies in metabolic-associated steatohepatitis-HCC (MASH-HCC) compared to viral-related HCC [72,115]. However, none of the phase III randomized controlled trials (RCTs) in advanced HCC have reported the percentage of patients with MASH-HCC. Consequently, indirect analysis of survival effects by etiology has been limited to non-viral HCC cases. A meta-analysis of three RCTs (IMbrave150, CheckMate 459, and Keynote-240) indicated that patients with viral-related HCC responded better to immunotherapies (HR, 0.64; 95% CI, 0.50–0.83) than those with non-viral-related HCC (HR, 0.92; 95% CI, 0.77–1.11; P=0.2) [115]. Following the publication of a subgroup analysis from the COSMIC-312 trial, a meta-analysis of four RCTs confirmed a significant difference in efficacy (P=0.01) [116]. When the HIMALAYA trial, which assessed a combination of two ICIs, was included in the meta-analysis (five RCTs), the difference remained significant, albeit less pronounced (P=0.046) [15]. These findings suggest that immunotherapies may be more effective in viral-related HCC than in other etiologies, supporting observations that MASH-HCC tumors have dysfunctional T cells, which may limit the effectiveness of ICIs [72].
However, these subgroup analyses are not statistically definitive and do not account for other prognostic factors. The term "non-viral etiologies" includes MASH-related, alcohol-related, idiopathic, and other metabolic causes, which complicates the analysis. These findings suggest that future studies should stratify participants based on etiology; however, dedicated prospective studies are necessary to determine the specific role of etiology. Although MASH-HCC is biologically distinct, the current clinical approaches remain consistent with those used for other non-viral etiologies, including alcohol-related HCC. Future trials should specifically identify cases of MASH-related HCC to better understand the impact of immunotherapies on the survival of this subgroup.
Conclusion
MASH is a significant global health issue and is projected to become the leading cause of HCC by 2030. The progression from MASH to HCC is influenced by molecular changes, the stage of fibrosis, the immune microenvironment, and the microbiome. Lifestyle changes are crucial for preventing MASLD progression, and surveillance in patients with MASH cirrhosis enables earlier detection and improves survival. Currently, MASH-HCC is managed similarly to other HCC etiologies, but comorbidities such as obesity and diabetes can complicate treatment.
Key unmet needs include identifying the molecular drivers of HCC in non-cirrhotic MASLD and developing preventive therapies. There is also a need for improved surveillance methods, particularly alternatives to ultrasound for obese patients, and for refining the selection of surgical candidates. It is crucial to report MASH-HCC outcomes separately in trials to facilitate better analysis; thus, it is recommended that MASH-HCC be specifically identified in clinical trials to enable more effective, personalized treatments. Additionally, further studies are required to understand MASH-HCC-related T-cell dysfunction and to identify biomarkers that predict treatment responses [117].