Introduction
The increasing incidence of precocious puberty is emerging as a significant medical and social issue worldwide [1,2]. A meta-analysis from 2020 reported a trend of breast development beginning approximately 0.24 years earlier every decade from 1977 to 2023 [3]. Additionally, there has been a notable decrease in the age of menarche from the 19th to the 20th century [4]. The onset of puberty is determined by both genetic and environmental factors [5,6]. Recent studies have highlighted that non-genetic lifestyle factors, such as adiposity, exposure to endocrine-disrupting chemicals (EDCs), and air pollution, might influence the timing of pubertal onset [7]. Furthermore, several epidemiological studies have found that exposure to ambient air pollution is linked to an earlier onset of menarche in girls [8,9].
Ambient air pollution consists of a mixture of particulate matter (PM) and gaseous pollutants that originate from both human activities and natural sources. This pollution primarily includes sulfur dioxide (SO2), nitrogen dioxide (NO2), carbon monoxide (CO), and ozone (O3). PM contains various components such as heavy metals, polycyclic aromatic hydrocarbons, and EDCs, all of which can interfere with the endocrine system [10,11]. When inhaled, ambient air pollution can enter the human bloodstream and be transported to various organs [12], leading to a range of health outcomes, including endocrine disruption [10]. PM can interact with estrogen receptors, triggering the release of kisspeptin, which in turn stimulates the secretion of gonadotropin-releasing hormone, thereby initiating the onset of puberty [13]. Moreover, fine particulate matter (PM2.5), with a diameter of less than 2.5 μm, as opposed to particulate matter (PM10), which has a diameter of less than 10 μm, can penetrate deeper into the body upon inhalation, potentially causing more severe adverse effects.
Currently, epidemiological studies that investigate the association between exposure to air pollution and pubertal development are limited, and their findings are inconsistent. It is important to note that, to date, no systematic review has been conducted on the relationship between exposure to air pollution and pubertal development.
Methods
Since this research did not involve any direct human participants or human-derived materials, it did not require approval from an institutional review board or the obtainment of informed consent.
We conducted a systematic review following the Preferred Reporting Items for Systematic Reviews and Meta-Analyses (PRISMA) 2020 guidelines (Fig. 1). The study protocol was registered with PROSPERO on September 19, 2023 (CRD42023465050). Revisions to the protocol were necessary as assessing the risk of bias and conducting a meta-analysis proved infeasible due to the heterogeneity among the included studies.
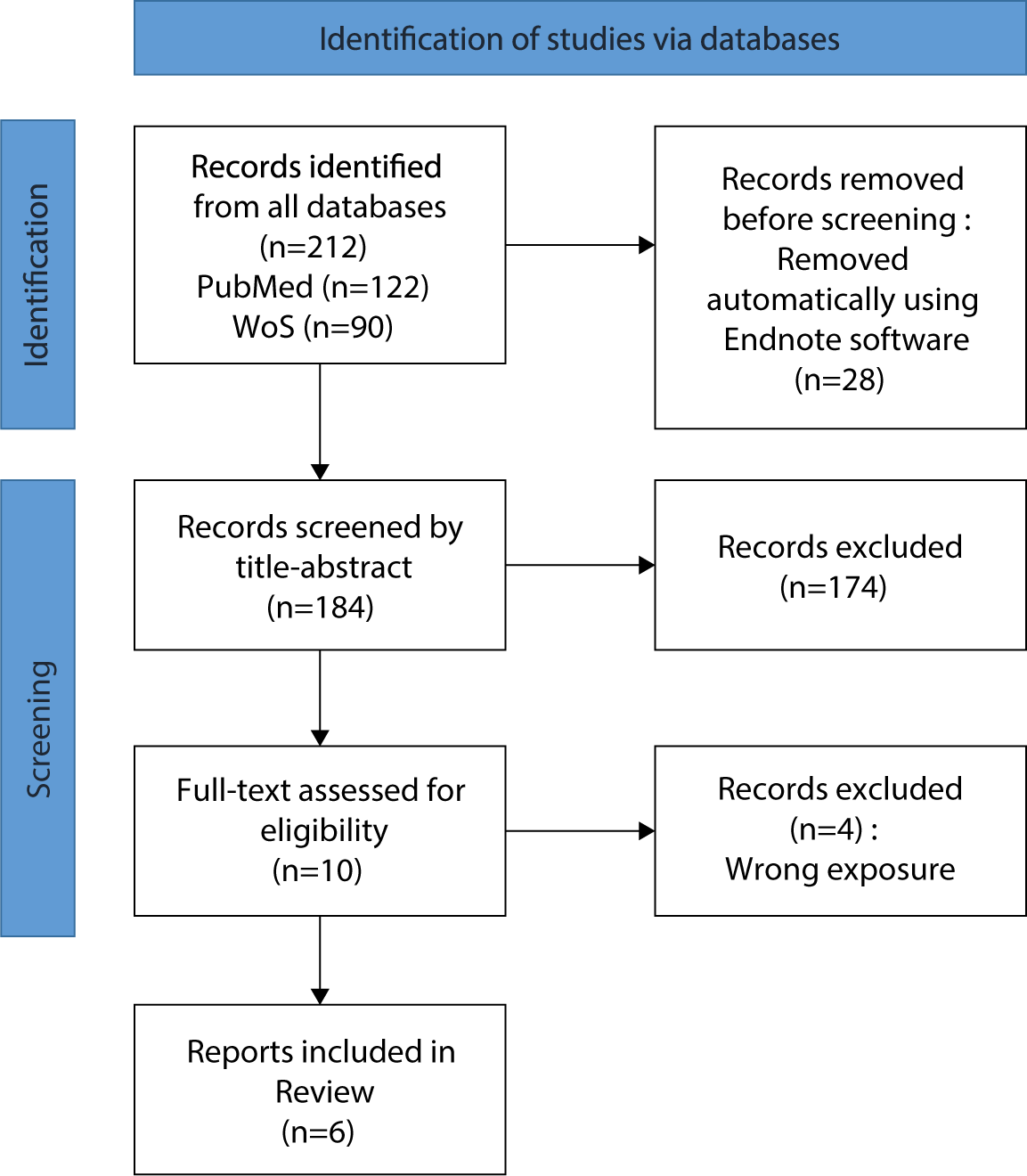
To investigate our systematic review, we defined the population, exposures, comparison, outcomes, and study designs (PECOS) parameters. The details of PECOS are as follows: a) population: infants, children, adolescents, or pediatric patients; b) exposure: air pollutants, such as PM2.5, PM10, SO2, NO, NO2, or O3; c) comparison: exposure to lower or higher levels of each specific type of air pollutants in the same population or in a control population; with provision of a measure of risk (e.g., relative risk [RR], OR, hazard ratio [HR], or mean difference [MD]); d) outcome: precocious puberty risk, early menarche risk, or pubertal development stage; and e) study design: human epidemiological studies, including prospective and retrospective cohort studies, case-control, and cross-sectional studies. We excluded abstracts, case reports, editorials, animal studies, in vivo studies, and commentaries.
We performed a systematic search of the 1) PubMed and 2) Web of Science (WoS) databases from their inception to August 23, 2023. Only articles in English were included.
The search terms for the search strategy can be found in Supplement 1. Duplicate articles were eliminated using EndNote and the manual method.
Two authors (RSL and EJM) independently reviewed the titles and abstracts and selected potentially eligible articles. Subsequently, the full texts of selected studies were examined by two authors (RSL and EJM) with the participation of other authors (JMO, KHK, JHL, HSK, and EHH) to address any disagreements and reach a consensus.
Two authors (RSL and JMO) extracted the following data of interest in a Microsoft Excel sheet: first author, year of publication, country, study design, follow-up period, sample size, population characteristics, exposure details, details on outcome assessment, and confounders. Furthermore, we also extracted measures of effect (i.e., RR, OR, HR, MD, and time ratio [TR]) for the association between air pollution and precocious puberty.
In our initial protocol for the systematic review, we planned to conduct a meta-analysis if at least three studies shared similar study designs, analysis methods, and effect sizes. However, a meta-analysis proved infeasible due to the limited number of studies (<3) and the diverse range of study designs, including case-crossover, cohort, and cross-sectional studies. Additionally, the outcomes varied among the studies, encompassing the risk of precocious puberty, the risk of early menarche, and stages of pubertal development. Given the heterogeneity among the included studies, we created a table to outline the characteristics of the studies and the relationships between the exposures and outcomes. As a result, we were unable to perform a meta-analysis and instead provided a descriptive summary.
Results
In our systematic search, we identified 184 studies out of the initial 212 (PubMed: 122 and WoS: 90), following the removal of duplicates. Of these, 10 full-text studies were assessed for eligibility, with 6 ultimately being included [8,9,14–17]. Fig. 1 illustrates the flow diagram of the study selection process. The studies that were excluded, along with the reasons for their exclusion, are detailed in Supplement 2.
Table 1 summarizes the characteristics of the six studies. Three studies were designed as cohorts [8,16,17], two were conducted as cross-sectional studies [9,15], and one was a case-crossover study [14]. These studies were conducted in China, Hong Kong, the USA, South Korea, Poland, and Germany. The sample sizes ranged from 437 to 4,074 participants, with follow-up durations spanning from 3 to 14 years. Five studies investigated exposure to ambient air pollutants such as PM2.5, PM10, SO2, NO, NO2, and O3 [8,9,14–16], whereas one study analyzed traffic-related metrics [17]. Yang et al. measured air pollutant levels using inverse distance weighting; Wronka et al. assessed air quality based on data from the chief inspectorate for environmental protection; Zhao et al. utilized a land-use regression model; Jung et al. relied on air monitoring network stations; Huang et al. gathered data from a monitoring station; and McGuinn et al. used annualized traffic data from the California Department of Transportation Highway Performance Monitoring System. The outcomes of these studies varied. Two studies [8,9] focused on the risk of early menarche as their primary outcome, with the age at menarche being self-reported. One study [14] examined the incidence of precocious puberty, defined by a professional pediatrician as the activation of the hypothalamic-pituitary-gonadal axis function and the onset of secondary sexual characteristics before the age of 8 in girls and 9 in boys. The remaining studies [15–17] assessed pubertal development at a specific age using Tanner staging or measured sex hormone levels.
Author | Country | Study design | Study period | Sample size | Age (years) | Sex Female (%) | Measured pollutants | Outcomes |
---|---|---|---|---|---|---|---|---|
Yang et al. [14] | China | Case-crossover | 2015–2021 | 2,201 | 7.47±1.24 | 96.6 | PM10, PM2.5, SO2, NO2, O3 | The risk of precocious puberty |
Wronka et al. [8] | Poland | Longitudinal cohort | 2015–2018* | 1,257 | 19–25 | 100 | PM10, PM2.5, benzene, SO2, NO | The risk of early menarche |
Zhao et al. [15] | Germany | Cross-sectional | 1995–2009† | 1,945 | 10 | 48.4 | PM10, PM2.5, NO2, O3 | Pubertal development at age 10 years assessed with estradiol and testosterone |
Jung et al. [9] | South Korea | Cross-sectional | 2010–2012 | 639 | 13–17 | 100 | PM10 | The risk of early menarche |
Huang et al. [16] | Hong Kong | Birth cohort | 1997–2008‡ | 4,074 | 9–12 | 47.5 | PM10, SO2, NO, NO2 | Pubertal stage at age 11 years assessed with Tanner stage |
McGuinn et al. [17] | USA | Longitudinal cohort | 2005–2012 | 437 | 6–8 | 100 | Traffic metrics | Pubertal stage at 6–8 years assessed with Tanner stage |
Table 2 summarizes the findings on the impact of air pollution on pubertal development, precocious puberty, and age at menarche across various studies. Four out of six studies [8,9,14,17] indicated that exposure to air pollution accelerates pubertal development stages and promotes precocious puberty. Jung et al. found that a 1 μg/m3 increase in PM10 was associated with a higher risk of early menarche (OR=1.08; 95% CI, 1.04–1.12) and accelerated age at menarche by 0.046 years (95% CI, −0.064 to −0.027) on a 1-year average. The authors reported that the results were consistent across the 2-year average (OR=1.06; 95% CI, 1.02–1.10; 0.038 years; 95% CI, −0.059 to −0.018) and 3-year model average (OR=1.05; 95% CI, 1.01–1.09; 0.031 years; 95% CI, −0.047 to −0.015). Wronka et al. found that the risk of early menarche (age below 11) was higher in the group living in areas with high PM levels. The ORs were calculated as 3.18 (95% CI, 2.29–4.69) for PM10 and 3.25 (95% CI, 2.34–4.8) for PM2.5. Yang et al. used a distributed lag nonlinear model to determine the OR of the lag effect of PM2.5 and PM10 on the incidence of precocious puberty. They reported that the most significant effects of PM2.5 and PM10 on precocious puberty were observed in lag 27 (OR=1.72; 95% CI, 1.01–2.92) and lag 16 (OR=1.95; 95% CI, 1.33–2.85), respectively. McGuinn et al. found that girls living within 150 m of a major road or highway had a higher likelihood of experiencing early pubarche (TR=0.96; 95% CI, 0.93–0.99), but not thelarche (TR=0.99; 95% CI, 0.97–1.02). The authors used accelerated failure time models, and calculated TRs, where a TR of <1.0 indicated an earlier age at pubertal development than the reference group. In contrast, Huang et al. reported that exposure to PM10 during the prenatal and infantile periods could delay thelarche. Exposure to PM10, SO2, NO, and NO2 was considered as z-scores for comparability, and the outcomes were the MD in Tanner stage per SD increment in each type of air pollutant. In girls, higher PM10 exposure in utero (MD: −0.05; 95% CI, −0.08 to −0.02) and in infancy (MD: −0.03; 95% CI, −0.06 to −1.2) was associated with a lower pubertal stage. In boys, higher SO2 exposure in utero (MD: −0.03; 95% CI, −0.05, −0.01) and during childhood (MD: −0.06; 95% CI, −0.08, −0.04) were associated with lower pubertal stage. Furthermore, higher NO2 exposure in utero (MD: −0.03; 95% CI, −0.04, −0.02) and during childhood (MD: −0.02; 95% CI, −0.04, −0.01) was associated with a lower pubertal stage. Zhao et al. found no statistically significant associations between air pollution exposure and pubertal development as assessed using serum sex hormone levels.
Author | Air pollutant exposure | Outcomes | Effect measure | Result with 95% CI | Adjusted variables | Average concentration of main air pollutants | |
---|---|---|---|---|---|---|---|
Yang et al. [14] | PM10 | The risk of precocious puberty | OR | 1.95 (1.33–2.85)† | Age, sex, SO2, NO2, CO, O3 | 69.77±41.07 μg/m3 | |
PM2.5 | 1.72 (1.01–2.92)† | 38.81±26.36 μg/m3 | |||||
Wronka et al. [8] | PM10 | Low* Medium High |
The risk of early menarche | OR | 3.18 (2.29–4.69)† | Urbanization, mother's education, father's education, number of siblings, financial conditions | NA |
PM2.5 | 3.25 (2.34–4.80)† | ||||||
Benzene | 1.11 (0.90–1.64) | ||||||
SO2 | 1.22 (1.01–2.14) | ||||||
NO | 1.47 (0.65–1.35) | ||||||
Zhao et al. [15] | PM10 | 10 μg/m3 increase in pollutant concentrations | Pubertal development at age 10 years assessed with estradiol and testosterone | OR | Female: 0.896 (0.379–2.122) Male: 0.821 (0.383–1.759) |
Age, sex, body mass index, secondhand smoke exposure, time spent outside and in front of a screen, physical activity level, season, and time of the blood sampling, household income, parental education, maternal age at birth, single parent status | 21.95±3.26 μg/m3 |
PM2.5 | Female: 0.163 (0.022–1.166) Male: 1.089 (0.156–7.605) |
14.76±2.13 μg/m3 | |||||
NO2 | Female: 0.892 (0.581-1.369) Male: 1.152 (0.768–1.728) |
22.03±3.86 μg/m3 | |||||
O3 | Female: 0.900 (0.605–1.339) Male: 0.830 (0.573–1.203) |
69.18±4.9 μg/m3 | |||||
Jung et al. [9] | PM10 | 1 μg/m3 increase in PM10 | The risk of early menarche | OR | 1.08 (1.04–1.12)† | Body mass index, city size, household income level, maternal age at menarche, second-hand smoke exposure at home | NA |
Huang et al. [16] | PM10 | SD increase in pollutants | Pubertal stage at age 11 years assessed with Tanner stage | Mean difference in Tanner stage | Female In utero: −0.05 (−0.08 to −0.02)† Infancy: −0.03 (−0.06 to −1.2)† |
Neighborhood and household income per person, mother's migration status, highest parental educational level, age, maternal age at birth, parity, maternal smoking | NA |
SO2 | Male In utero: −0.03 (−0.05 to −0.01)† Childhood: −0.06 (−0.08 to −0.04)† |
||||||
NO | Statistically insignificant | ||||||
NO2 | Male In utero: −0.03 (−0.04 to −0.02)† Childhood: −0.02 (−0.04 to −0.01)† |
||||||
McGuinn et al. [17] | Traffic metrics | Distance to road (meters) | Pubertal stage at 6−8 years assessed with Tanner stage | TR | Pubarche: 0.96 (0.93–0.99)† Thelarche: 0.99 (0.97–1.02) |
Race/ethnicity, household income, girl's cotinine level | NA |
PM, particulate matter; SO2, sulfur dioxide; NO2; nitrogen dioxide; CO, carbon monoxide; O3, ozone; TR, time ratio; NA, not applicable.
Discussion
In this systematic review, we found that four out of six studies indicated a relationship between increased exposure to air pollution and earlier onset of puberty [8,9,14,17]. One study produced inconclusive results [15], while another suggested that exposure to air pollutants might delay the onset of thelarche [16].
There has been no prior systematic review examining the effects of air pollution exposure on pubertal development and precocious puberty. The studies we included employed various research designs, such as cohort, cross-sectional, and case-crossover studies, featured different sample sizes, and tracked participants over varying lengths of time. These studies also investigated different exposures and outcomes and utilized a range of methods to measure exposure. We recognize that this diversity in study design could account for the inconsistent results regarding the impact of air pollution on precocious puberty. Nevertheless, the authors are inclined to believe that air pollution adversely affects precocious puberty, based on the accumulating evidence that air pollutants influence pubertal development through various mechanisms, which are not yet fully understood.
When chemicals and heavy metals with endocrine-disrupting properties are released into the air from industrial emissions, vehicle emissions, and waste combustion, they can bind to PM [18]. Polycyclic aromatic hydrocarbons and heavy metals in PM, particularly from fossil fuel combustion, are recognized as endocrine disruptors due to their ability to activate aryl hydrocarbon, androgen, or estrogen receptors [19]. PM can act on estrogen receptors, triggering the release of kisspeptin, which subsequently initiates the secretion of gonadotropin-releasing hormone, thus starting the onset of puberty [13]. Epigenetic disruption caused by PM is a potential mechanism for triggering puberty through neuroendocrine components [20]. Moreover, PM can induce oxidative stress and systemic inflammation upon entering the respiratory tract [21]. Endocrine disruptors attached to PM influence hormone synthesis in endocrine glands or disrupt hormone transport to target organs. Research using mixtures of EDCs found in indoor air samples has shown that these compounds exhibit estrogenic and androgenic activities when tested in in vitro assay systems [22]. There is increasing evidence that certain EDCs are associated with various human health issues, such as reproductive problems in both females and males, and precocious puberty in children, as indicated in several previously reported systematic reviews [23–28].
Although the exact mechanisms connecting air pollutants and puberty onset remain unclear, the presence of EDCs in air, including PM, and their potential impact on puberty are areas that require active research.
These studies stand out for their pioneering research into the relationship between exposure to air pollutants and pubertal development. McGuinn et al. [17] conducted the first study to explore the link between early life proximity to traffic and pubertal development within a multiethnic cohort. Huang et al. utilized a population-representative birth cohort and gathered clinical data on pubertal stages. Jung et al. [9] analyzed data from the fifth Korea National Health and Nutrition Examination Study, a representative sample of the South Korean population, and found consistent results across various models after making adjustments. Wronka et al. [8] carried out the inaugural study in a European country investigating the connection between air pollutant exposure and early menarche. Zhao et al. [15] also drew on data from two relatively large birth cohorts, with numerous relevant covariates available for adjustment. Yang et al. [14] employed a case-crossover study design and a distributed nonlinear model to evaluate the association between PM10 and PM2.5 levels and the risk of precocious puberty. Zhao et al. [15] and Jung et al. [9] considered body mass index as a confounding factor, acknowledging that nongenetic lifestyle factors such as adiposity can influence the onset of puberty.
Nonetheless, the included studies have several limitations. First, exposure to air pollutants was estimated using data averaged for specific geographic areas, and the limited number of air quality monitoring stations may not provide a precise representation of individual exposure. Consequently, exposure misclassification and a lack of individual-level data are possible issues. Second, some studies were susceptible to recall bias, particularly those relying on self-reported data such as age at menarche. Additionally, most studies focused on single pollutant exposure, whereas in reality, humans are exposed to a complex mixture of air pollutants [29]. Moreover, the study designs varied, encompassing different exposures to air pollutants, and the outcomes included the incidence of precocious puberty, age at menarche, and pubertal stage at a specific age. This heterogeneity in study design and outcomes made it infeasible to conduct a meta-analysis and evaluate the risk of bias.
Future researchers should consider conducting prospective birth cohort studies to assess the long-term consequences of prenatal and postnatal exposure to air pollution. This is crucial because EDCs can function as obesogens during pregnancy, potentially altering fetal programming [30], and air pollution may have similar effects. Furthermore, to accurately assess individual exposure to air pollution, it is feasible to use advanced technologies and methods, such as personal monitoring devices. Expanding the study population to include diverse socioeconomic groups and geographical locations can enhance the generalizability of the findings. In vitro research is also necessary to understand the biological mechanisms underlying the association between air pollution and pubertal development. Additionally, research should focus on developing prevention policies and interventions aimed at mitigating the impact of air pollution. Air pollution is a global concern, and international collaboration among researchers and institutions worldwide can lead to a more comprehensive understanding of its effects on health.
To the best of our knowledge, this is the first systematic review to provide evidence of the impact of air pollution exposure on precocious puberty and pubertal development. Additionally, all review processes underwent peer review, adhering to the PRISMA guidelines. However, due to the heterogeneity among the studies, it was not feasible to conduct a meta-analysis to evaluate the combined effect of air pollution on the risk of precocious puberty. Future research on this topic is necessary, and as more studies become available, we aim to gather sufficient evidence to conduct a meta-analysis.
The evidence suggests that exposure to air pollution may lead to an earlier onset of puberty, although the results of studies have been inconsistent. To address this, further longitudinal studies are needed that accurately assess individual exposure to multiple air pollutants over extended periods. It is crucial to promote policies aimed at reducing exposure to air pollution. Additionally, sharing international data and conducting collaborative studies could provide valuable insights for developing preventive policies concerning exposure to air pollutants.